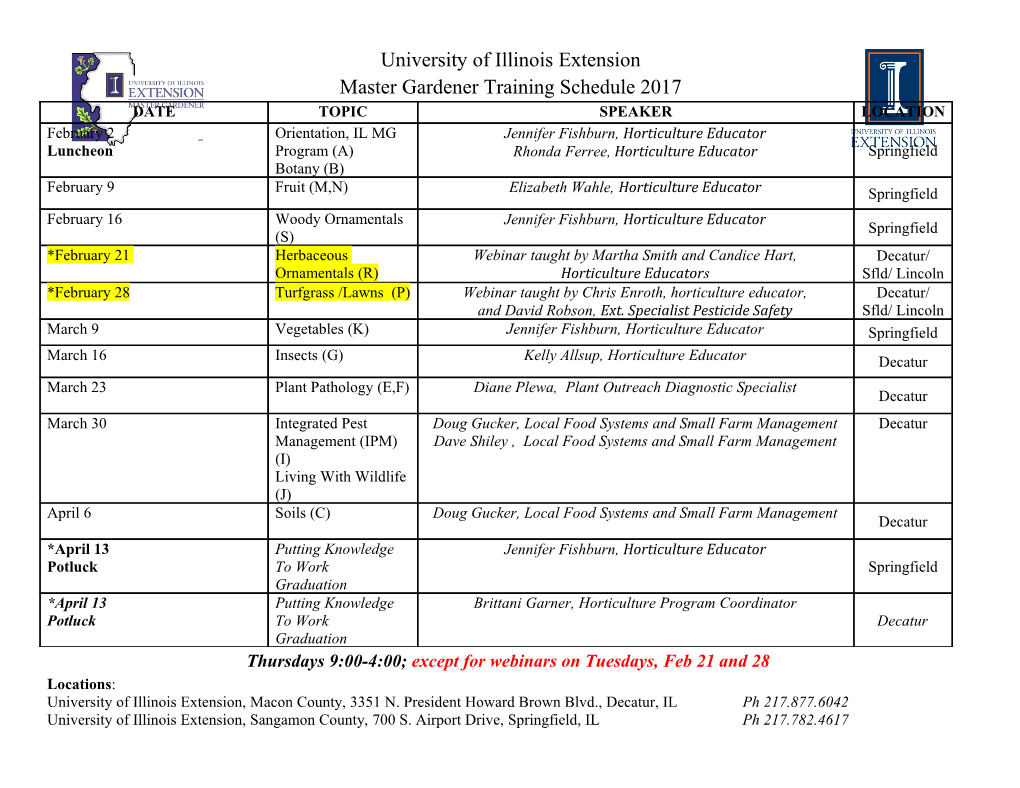
Active Electrokinetic Transport Control in a Nanofluidic Device with Embedded Surface Electrodes DISSERTATION Presented in Partial Fulfillment of the Requirements for the Degree Doctor of Philosophy in the Graduate School of The Ohio State University By Marie J. Fuest Graduate Program in Mechanical Engineering The Ohio State University 2016 Dissertation Committee: Professor Shaurya Prakash “Advisor” Professor Carlos Castro Professor A.T. Conlisk Professor Steven Ringel Copyright by Marie J. Fuest 2016 Abstract Since the 1990s, lab-on-a-chip (LoC) systems, or miniaturized devices that perform several unit operations that typically require bench top sized equipment on an integrated platform have been developed for applications in biotechnology, chemical and biological reactors, energy conversion systems, separation and filtration systems, and medical, pharmaceutical, and environmental monitoring. The success of LoC technology relies on the ability to manipulate ions and molecules in increasingly small volumes of fluid (nL and less). With decreasing critical length scales for devices made possible by advances in microfabrication and nanofabrication, the device surface-area-to volume ratio has made the role of surface properties critical to operation and novel functionality of many of these devices. Of the various surface properties, surface charge presents a unique parameter with broad applicability for engineering new device functionalities. Gated nanofluidic devices, geometrically analogous to semiconductor field-effect transistors, feature a nanofluidic channel with a “gate” electrode embedded in the nanochannel wall, providing an active, tunable region for manipulation of local surface charge. Specifically, an independently controlled potential applied to the embedded gate electrode allows systematic manipulation of the surface charge density at the dielectric-electrolyte interface. Fabrication protocols for gated nanofluidic devices with sub-20 nm critical length scales were developed that rely on UV lithography, wet etching, ii and oxygen plasma bonding techniques. Active control over ionic transport through the nanochannel was monitored by changes in current as a function of gate potential, electrolyte type, electrolyte concentration, and solution pH. The measured current was referenced with respect to the ungated or intrinsic case, or the case when only an axial potential was applied with no gate potential. In this dissertation, it was experimentally verified that the gate electrode additionally alters the electric field in the nanochannel by modifying the potential in the nanochannel near the gate electrode/dielectric/solution interface. In the surface charge governed transport regime, the gate electrode was used to switch off the measured current for a fixed axial potential, where repeatable on/off switching was demonstrated. Local variation of surface charge by the gate electrode on transport of multivalent aqueous electrolytes and electrolyte mixtures, which form the basis for biological or practical applications, was investigated as a function of cation type. In agreement with previous anomalous transport reports, no significant difference was observed for the intrinsic nanochannel conductance of KCl compared to NaCl. Conductance decreased for MgCl2 and CaCl2 at concentrations typically associated with surface charge governed transport for monovalent electrolytes, suggesting a decrease in the total surface charge due to divalent cation adsorption at the negatively charged walls. Data from KCl and 2+ CaCl2 electrolyte mixtures clearly indicated that Ca in the mixture is the dominating ion as determined by a decrease in surface charge density when CaCl2 was added and a surface charge density that was independent of KCl concentration for %CaCl2 > 25%. Cation adsorption to the charged walls regulates the surface charge density thus limiting iii the ability of the gate electrode to alter the net surface charge and consequently modulate nanochannel conductance as a function of cation type. iv Dedication This document is dedicated to Ciana Marie Pinti. v Acknowledgments I would like to thank my advisor, Shaurya Prakash, for his guidance and support throughout my PhD. I would like to thank Professor A.T. Conlisk for his insight and useful suggestions that have helped guide the work in this dissertation. I would also like to thank my committee members, Professor Carlos Castro and Professor Steven Ringel, for their useful insights into my work. I would like to thank the staff at Nanotech West laboratories for their assistance with equipment during fabrication and characterization of nanofluidic device. I want to thank all of my fellow lab mates for their hard work and the many things that I have learned from them over the years. Special thanks to Caitlin Boone, whose dedication and enthusiasm have made her a true pleasure to work with over the past year. I would also like to thank Kaushik Rangharajan for his work developing COMSOL models to describe ionic transport behavior in the nanofluidic device presented here. Past and current undergraduate researchers, Neil Ramirez, David Horner, Kyle Wellmerling, and Bowen Wang, who assisted with experimental setup and worked on projects related to this work, are acknowledged for their hard work, creativity, and insightful questions. I would also like to thank Professor Harvey Zambrano for useful conversations that gave me a fresh perspective at different stages of this project. Eugene Sosnov is acknowledged for providing me with initial training in clean room processes. Special thanks to Karen vi Bellman Lowe for helping me transition from Physics to Mechanical Engineering, for her unconditional support, and her invaluable friendship. Finally, I would like to thank my parents for their unconditional love and support and their reassurance throughout my academic career. Special thanks to Frederik Fuest for his encouragement and support. I would like to acknowledge partial financial support for this research from the US Army Research Office (ARO) through grant number W911NF09C0079 and the National Science Foundation through grant CBET-1335946. I would also like to thank the National Science Foundation for support through their Graduate Research Fellowship Program (GRFP) and the Ohio State University for their support through the Distinguished University Fellowship for my first year and dissertation year. vii Vita May 2006 .......................................................Charles F. Brush High School 2010................................................................B.S. Physics, John Carroll University 2014................................................................M.S. Mechanical Engineering, Ohio State University 2010 to present ..............................................Graduate Research Fellow, Department of Mechanical Engineering, The Ohio State University Publications 1. Marie Fuest, C. Boone, A.T. Conlisk, and S. Prakash; Cation Dependent Surface Charge Regulation in Gated Nanofluidic Devices. 2015, (submitted). 2. K. K. Rangharajan, Marie Fuest, A.T. Conlisk, S. Prakash; Transport of Multicomponent, Multivalent Electrolyte Solutions across Nanocapillaries. Microfluidics and Nanofluidics, 2015, (in review) 3. S. Prakash, H. A. Zambrano, Marie Fuest, C. Boone, E. Rosenthal-Kim, N. Vasquez, and A.T. Conlisk; Electrokinetic Transport in Silica Nanochannels viii with Asymmetric Surface Charge. Microfluidics and Nanofluidics, 2015, 19 (6), 1455-1464. 4. Marie Fuest, C. Boone, A.T. Conlisk, and S. Prakash; Cation Dependent Transport in a Field Effect Nanofluidic Device. Technical Digest of the 18th International Conference on Solid-State Sensors, Actuators and Microsystems Transducers 2015, Anchorage, Alaska, 6/21-25/2015 Transducers Research Foundation, Cleveland (2015). 5. Marie Fuest, C. Boone, K. K. Rangharajan, A.T. Conlisk, S. Prakash; A Three- State Nanofluidic Field Effect Switch. Nano letters, 2015, 15 (4), pp 2365–2371. 6. Marie Pinti (Fuest), H.A. Zambrano, K.K. Rangharajan, B. Wang, A.T. Conlisk, and S. Prakash; Active surface charge control for artificial ion pumps, Technical Digest of the Hilton Head Workshop 2014: A Solid-State Sensors, Actuators and Microsystems Workshop, Hilton Head, South Carolina, 6/8-12/2014 Transducers Research Foundation, Cleveland (2014), pp. 315-317. 7. Marie Pinti (Fuest), T. Kambham, B. Wang, S. Prakash; Fabrication of centimeter long, ultra-low aspect ratio nanochannel networks in borosilicate glass substrates. Journal of Nanotechnology in Engineering and Medicine, 2013, 4, pp. 021003 (1-7). 8. Marie Pinti (Fuest), S. Prakash. (2013). Fabrication of Hybrid Micro- Nanofluidic Devices with Centimeter Long Ultra-Low Aspect Ratio Nanochannels. 2013 Proceedings of the 2013 ASME International Mechanical Engineering Congress and Exposition, San Diego, California. ix 9. H A Zambrano, Marie Pinti (Fuest), A. T. Conlisk, S Prakash; Electrokinetic transport in a water–chloride nanofilm in contact with a silica surface with discontinuous charged patches. Microfluidics and Nanofluidics, 2012, 13(5), 735-747. 10. V. V. Swaminathan, L. R. Gibson II, Marie Pinti (Fuest), S. Prakash, P. W. Bohn, M.A. Shannon; Ionic transport in nanocapillary membrane systems. Journal of Nanoparticle Research, 2012, 14(8), 951-965. 11. S. Prakash, Marie Pinti (Fuest), K. Bellman; Variable cross-section nanopores fabricated in silicon nitride membranes using a transmission electron microscope. Journal of Micromechanics and Microengineering, 2012, 22 067002.
Details
-
File Typepdf
-
Upload Time-
-
Content LanguagesEnglish
-
Upload UserAnonymous/Not logged-in
-
File Pages367 Page
-
File Size-