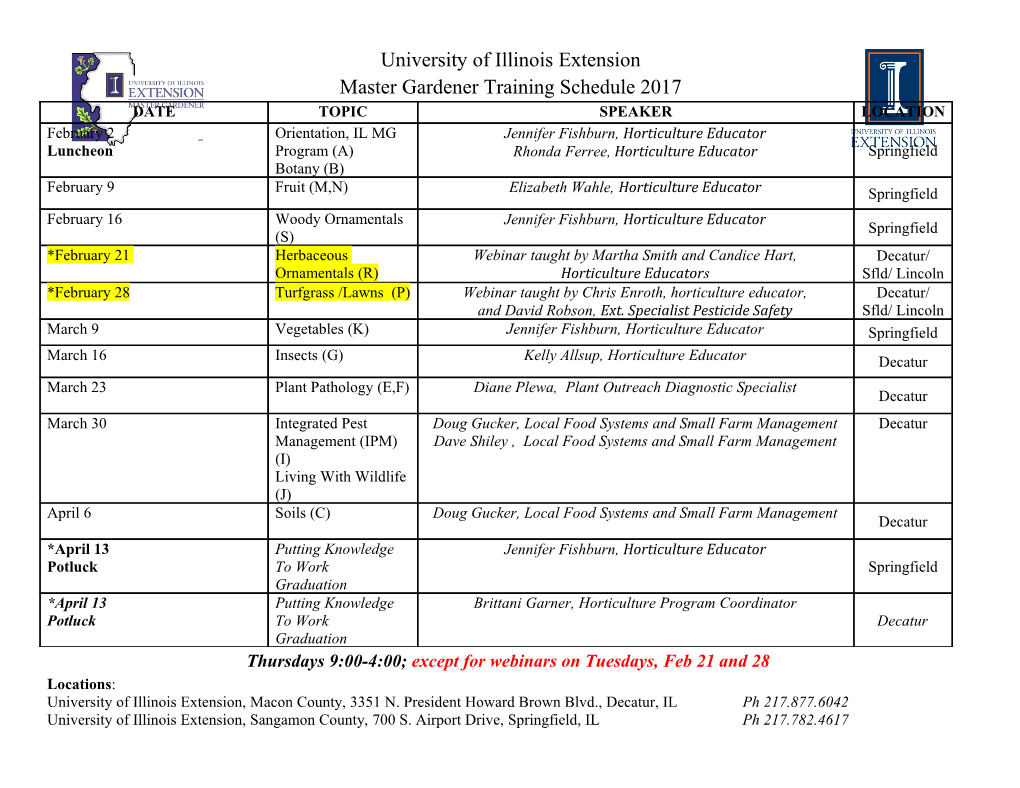
SPATIAL AND TIME RESOLVED STUDY OF TRANSIENT PLASMA INDUCED OH PRODUCTION IN QUIESCENT CH4-AIR MIXTURES by Charles Cathey A Dissertation Presented to the FACULTY OF THE GRADUATE SCHOOL UNIVERSITY OF SOUTHERN CALIFORNIA In Partial Fulfillment of the Requirements for the Degree DOCTOR OF PHILOSOPHY (ELECTRICAL ENGINEERING) December 2007 Copyright 2007 Charles Cathey Abstract This thesis presents an experimental study into the utility of transient plasma ignition (TPI) of hydrocarbon fuel-air mixtures and the basic physics behind said phenomena. Transient plasma has several advantages over traditional spark ignition, consistently demonstrating reductions in ignition delay and extended lean burn capability. It is postulated that high energy electrons generated during the electrical discharge are responsible for electron impact dissociation, ionization, and fragmentation of molecules in the medium resulting in radical formation that drives the combustion process. The experiments described in this thesis illustrate TPI’s effects and attempt to understand the underlying physics by looking at transient plasma induced production of radicals, ignition, and flame propagation. Using optical diagnostic techniques the ability of a transient plasma to populate a cylindrical discharge volume with the hydroxyl radical (OH) is analyzed during combustion of stoichiometric quiescent CH4 – air mixtures. Ground state OH production is analyzed using planar laser induced fluorescence (PLIF). Broadband transitions of OH near 309 and 314 nm were also used to monitor transient plasma production of OH* via optical emission spectroscopy (OES). A high speed camera was used to image ignition and flame propagation in the chamber, providing spatial and temporal resolution over the entire combustion event. Transient plasma successfully ignited the CH4 - air mixture, populating the discharge volume with radicals. Mean OH number densities produced by the x discharge were found to reach ~ 4·1014 molecules – cm-3 and decay within 100 µs of the plasma. Ignition under these conditions was found to occur approximately 1 ms after the discharge along the anode, creating a cylindrically expanding flame front. TPI was found to be a truly volumetric ignition source creating multiple ignition kernels whose proximity to the anode is consistent with the region of highest field and thus maximum radical density. Additionally the rapid decay of the initial OH (as seen by the pump beam) with respect to the time of ignition suggests that the anode itself may have an important role in sustaining and enhancing combustion kinetics. xi Chapter 1 Introduction to Transient Plasma Introduction There is a great interest in the combustion community for non-equilibrium (Te >> Tgas) plasma devices that accelerate ignition and stabilize combustion. Specific applications are in the development and implementation of non-equilibrium plasma ignition sources for supersonic and hypersonic aircraft and low emission automobiles. Using the non-equilibrated plasma in first stages of arc development for ignition, subsequently known as transient plasma ignition (TPI), great reductions in ignition delay a factor of 2 to 9, and the extension of the lower flammability limit for a variety of fuels has been demonstrated. Equilibrated Atmospheric Pressure Plasmas The most familiar ignition methodology uses an equilibrated (Te = Ti) or nearly equilibrated plasma in the form of an arc or spark which is commonly used in both automobile and aircraft engines. For this type of discharge, ignition is achieved by local heating of the gas, which increases the dissociation rate, and reactions of chain branching and propagation. The arc discharge is the classic example of a quasi-equilibrated plasma, even though they are often far from equilibrium. The arc cathode has a relatively low cathode fall voltage of about 10 eV, corresponding to the ionization potential. Additionally electron emission is via thermionic and field effects. The high current typical in arcs results in the cathodes and the gas being subjected to large amounts of joule heating. The high amount of current produced leads to erosion and evaporation 1 of the cathode. However, there is an alternate way to ignite the mixture in the form of non-equilibrated plasmas. Non-equilibrated Atmospheric Pressure Plasmas The basic difference between equilibrated (thermal) plasmas and non- equilibrated (non-thermal) plasmas is in the ionization mechanisms of the two processes. The ionization process in non-equilibrated plasmas is dominated by electrons impacting with “cold” non-excited atoms and molecules. Additionally in non equilibrated plasmas the majority of the energy goes into creating highly energetic electrons instead of heating of the gas. These electrons produce excited species through impact dissociation, excitation, and ionization of background gas molecules in the system. Another area of distinction is that non-equilibrated plasmas seem to be very selective as to the allowed plasma chemical reactions. A common application would be treatment of exhaust gasses, where the radicals created via the non thermal plasma, are able to oxidize, or decompose the pollutant molecules.1 Whereas pollution control with a thermal system (plasma torch) heat the entire gas system in order to destroy the pollutants. Electric Breakdown of Gases The subsequent sections describing the breakdown process are based on Fridaman and Razier.2,3 When an electric field is applied some primary electrons near the cathode will form and drift towards the anode. As the electrons drift towards the anode, the gas will ionize and an avalanche will form. Electrical breakdown in a gas is usually preceded by an electron avalanche, where a 2 multiplication in the number of primary electrons occurs over in a cascade of ionization. The ionization in an avalanche is described by the Townshend ionization coefficient α, which refers to the electron production per unit length. The Townshend ionization coefficient is related to the ionization rate coefficient ki (E/n0) and electron drift velocity vd by: ν i 1 1 ki (E / n0 ) α = = ki (E / n0 )n0 = (1-1) ν d ν d µe E / n0 Where vi is the ionization frequency, µe is the electron mobility, and E/no is the applied electric field over the neutral gas density, also called the reduced electric field. Each primary electron produced near the cathode creates exp(αd)-1 positive ions in the gap. The total electronic current is the electronic current at the anode, because of the absence of ionic current, which gives the Townsend formula: i exp(αd) i = 0 (1-2) 1− γ[exp(αd) −1] γ is the secondary electron emission coefficient, and depends on the cathode material, sate of the surface, type of gas, and E/n0. The current will not sustain as long as the denominator in equation (1-2) is positive. As the applied electric field is increased the Townsend α coefficient will also increase and eventually will push the denominator to zero, and transition to self sustained current, or breakdown occurs. Thus the simplest breakdown condition can be expressed using (1-2) as: ⎛ 1 ⎞ γ[exp(αd) −1] = 1 , or αd = ln⎜ +1⎟ (1-3) ⎝ γ ⎠ It is convenient to re-write equation 1 in terms of α in the following way: 3 α ⎛ B ⎞ = Aexp⎜− ⎟ (1-4) p ⎝ E / p ⎠ Where A and B are experimental parameters. In air A = 15 1/ cm Torr, and B = 365 V/cm Torr. Combining equations (1-3) and (1-4) gives what is commonly called the Paschen curve. B( pd) V = (1-5) break C + ln( pd) ⎛ ⎞ ⎜ ⎟ ⎜ A ⎟ Where C = ln⎜ ⎟ , which accounts for the weak influence of secondary ⎜ ⎛ 1 ⎞ ⎟ ⎜ ln⎜ +1⎟ ⎟ ⎝ ⎝ γ ⎠ ⎠ electron emissions. The Townshend breakdown formula is only applicable for pd < 4000 Torr cm, or at atmospheric conditions, d < 5 cm. In larger gaps, and higher pressures spark breakdown occurs. While the Townsend breakdown is quasi-homogenous, spark breakdown occurs across a narrow channel, is not directly related to electrode phenomena, and has very high current currents. The method of spark breakdown is based on the concept of the streamer. Electron Avalanches The electron avalanche that occurs when an electric field is applied is a critical step in the breakdown process. In electronegative gasses, like air, electrons will have a tendency to attach to oxygen molecules. Accounting for electron 4 attachment, the rate of change of the total number of electrons Ne, positive N+ and negative N- ions in an avalanche moving in the direction x is: dN dN dN e = ()α − β N , + = αN , − = βN (1-6) dx e dx e dx e Where α is the Townshend ionization coefficient and β is the Townsend attachment coefficient. Assuming that the avalanche starts from only the primary electrons the number of charged particles can be found using equation 6 as: α β N = exp[(α − β )x], N = (N −1) , N = (N −1) (1-7) e + α − β e − β −α e The electrons in the avalanche will move in the x direction with the applied electric field at their drift velocity, vd = µeEo. However, free diffusion (De) of the electrons will also be taking place, spreading the electrons out radially (direction r) from the x direction. Accounting for drift and diffusion, the electron density in the avalanche is given as: 2 2 1 ⎡ (x − µe E0t) + r ⎤ ne (x,r,t) = exp⎢− + (α − β )µe E0t⎥ (1-8) 3 / 2 4D t ()4πDet ⎣⎢ e ⎦⎥ Equation (1-8) shows that the avalanche electron density will decrease with distance from x as diffusion occurs. Taking into account the Einstein relation between electron mobility and free diffusion the avalanche head radius, rA will also increase with time, and distance of propagation along x (x0). x0 4Te rA = 4Det = 4De = x0 (1-9) µe E0 eE0 5 Using equation (1-8) the space distribution of charge for the negative and positive ion densities during avalanche propagation are: t t n (x,r,t) = αµ E n (x,r,t')dt' , n (x,r,t) = βµ E n (x,r,t')dt' (1-10) + ∫ 0 0 e − ∫ 0 0 e 0 0 A simplification for positive ion space distribution can be found using equations (1- 10) and (1-8) for densities close to the x axis, in the absence of attachment as t → ∞.
Details
-
File Typepdf
-
Upload Time-
-
Content LanguagesEnglish
-
Upload UserAnonymous/Not logged-in
-
File Pages151 Page
-
File Size-