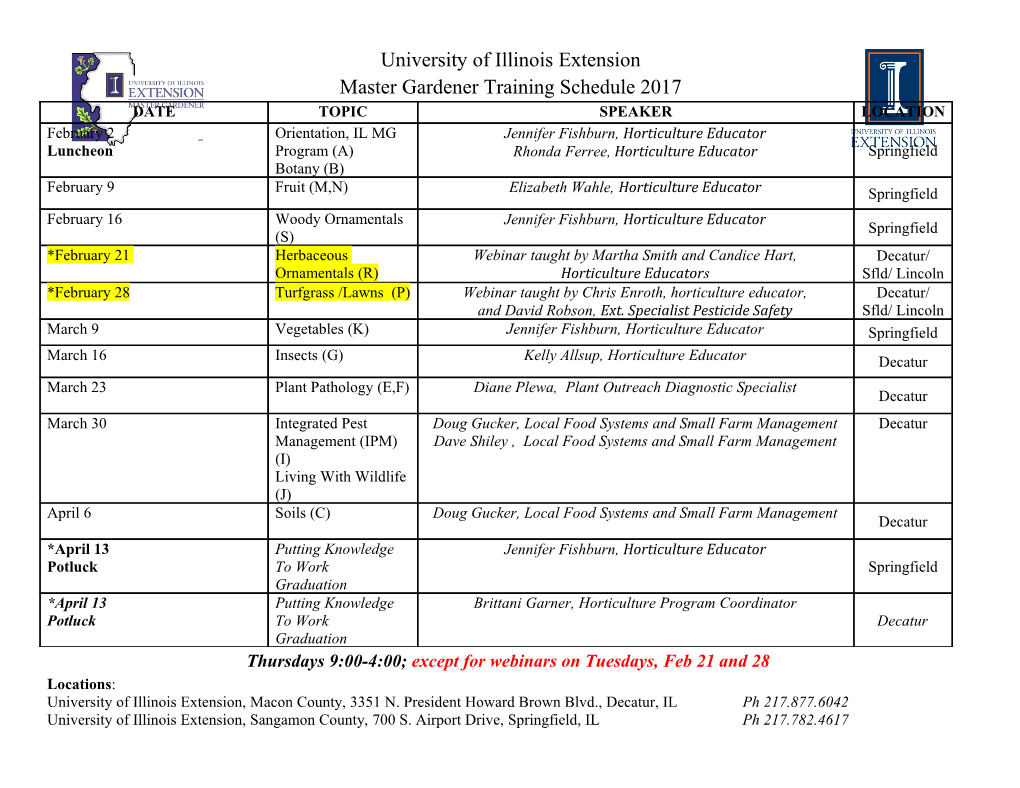
Review April0???Tansley 2008 review review TansBlackwellOxford,NPHNew0028-646X1469-8137© The Phytologist Authors UK Publishing (2008). Ltd Journal compilation ley© New Phytologist (2008) reviewTansley review Branching out in new directions: the control of root architecture by lateral root formation Author for correspondence: C. Nibau*, D. J. Gibbs* and J. C. Coates J. C. Coates School of Biosciences, University of Birmingham, Edgbaston, Birmingham B15 2TT, UK Tel: +44 121 414 5478 Fax: +44 121 414 5925 Email: [email protected] Received: 21 December 2007 Accepted: 14 March 2008 Contents Summary 595 V. Transcriptomic studies to identify potential new regulators of lateral root development 608 I. Background 595 VI. Conclusions and future challenges 608 II. Formation of lateral roots 596 Acknowledgements 608 III. Endogenous factors regulating the stages of lateral root development 597 References 609 IV. Plasticity: modification of lateral root development by the environment 603 Summary Key words: abiotic stress, biotic stress, Plant roots are required for the acquisition of water and nutrients, for responses to lateral root development, nutrients, plant abiotic and biotic signals in the soil, and to anchor the plant in the ground. Controlling hormones, root system architecture, plant root architecture is a fundamental part of plant development and evolution, transcriptomics. enabling a plant to respond to changing environmental conditions and allowing plants to survive in different ecological niches. Variations in the size, shape and surface area of plant root systems are brought about largely by variations in root branching. Much is known about how root branching is controlled both by intracellular signalling pat- hays and by environmental signals. Here, we will review this knowledge, with particular emphasis on recent advances in the field that open new and exciting areas of research. New Phytologist (2008) 179: 595–614 © The Authors (2008). Journal compilation © New Phytologist (2008) doi: 10.1111/j.1469-8137.2008.02472.x structural anchor to support the shoot. The root system I. Background communicates with the shoot, and the shoot in turn sends A plant’s root system is the site of water and nutrient uptake signals to the roots. A plant root system initially consists of a from the soil, a sensor of abiotic and biotic stresses, and a primary root (PR) formed during embryogenesis that has dividing cells in a meristem at its tip. As the seedling develops, *These authors contributed equally to this work. certain other cells within the PR acquire the capability to www.newphytologist.org 595 596 Review Ta n s l e y re v ie w hair development, primary root (PR) growth and AR formation. However, an extensive analysis of how these structures are controlled is outside the scope of the present review and the reader is referred to several other excellent reviews (Dolan & Costa, 2001; Carol & Dolan, 2002; Scheres et al., 2002; Casson & Lindsey, 2003; Hochholdinger et al., 2004; Samaj et al., 2004; Serna, 2005; Scheres, 2007). Moreover, colonization of certain plant roots by symbiotic bacteria or fungi leads to the formation of modified LRs (root nodules, mycorrhizas or proteoid roots) that carry out specialized functions such as nutrient acquisition (Oldroyd & Downie, 2004, 2006; Autran et al., 2006). In addition to signals that regulate many components of Fig. 1 Components of the root system. (a) A typical dicot RSA (and sometimes also shoot development), there is (e.g. Arabidopsis) seedling root system, consisting of a primary mounting evidence that some signalling networks are specific root (PR) originating from the embryo, lateral roots (LR) branching for LR formation (Rogg et al., 2001; Hochholdinger et al., out from the PR during seedling development, and root hairs 2004; Loudet et al., 2005; Coates et al., 2006), potentially (RH) that originate from PR epidermal (Epi) cells (shown at highlighting novel strategies for manipulating root branching higher magnification to the right (inset)). Ultimately, the LRs will undergo higher-order branching to form secondary and in crop plants. tertiary LRs. Adventitious roots (AR) form at the shoot–root junction. Because of the major contribution they play in the control (b) A typical cereal (e.g. rice, maize) seedling root system consisting of RSA, this review focuses on LRs: how they arise and develop. of a primary root (PR) originating from the embryo, seminal roots (SR) It will pay particular attention to recent molecular and ‘omic’ that originate postembryonically close to the top of the primary root, developments that highlight the huge variety of genes, and crown roots (CR) that originate from the stem. PR, SR and CR all form LR and undergo higher-order branching. proteins and mechanisms that interact together to coordinate a process so central to plant development and survival. divide, eventually forming new roots, called lateral roots (LRs) II. Formation of lateral roots (Fig. 1a). These branch out from the PR, greatly increasing the total surface area and mechanical strength of the root In flowering plants and gymnosperms, LRs initiate from a system and allow the plant to explore the soil environment. specialized cell layer in the PR called the pericycle. The Ultimately, millions of higher-order root branches can form, pericycle is the outermost cell layer of the vascular cylinder resulting in hundreds of miles of root system in a small area and consists of two distinct cell types corresponding to the of soil (Dittmer, 1937). New roots, called adventitious roots underlying vasculature (Dubrovsky & Rost, 2005; Parizot (AR), can also be formed postembryonically at the shoot–root et al., 2008; Fig. 2a,b). In Arabidopsis and most other dicots, junction, optimizing the exploration of the upper soil layers LRs are formed only from pericycle cells overlying the (Fig. 1a). In cereals such as rice and maize, root structure developing xylem tissue (the xylem pole pericycle) (Fig 2b). In becomes more complex, with the formation of additional other species, particularly cereals such as maize, rice and wheat, shoot-borne and postembryonic roots, which in turn undergo LRs arise specifically from the phloem pole pericycle, with higher-order branching (Hochholdinger et al., 2004; Hoch- additional contributions from the endodermis (De Smet et al., holdinger & Zimmermann, 2008; Fig. 1b). 2006a; Hochholdinger & Zimmermann, 2008; Fig. 2b). The root system architecture (RSA) of plants varies hugely Insights into the evolution of multicellular, branched root between species and also shows extensive natural variation systems come from ‘ancient’ plants. In a vascular nonseed within species, reflecting the plethora of environments in plant, the fern Ceratopteris, LRs arise from the endodermis which plants can grow (Cannon, 1949; Loudet et al., 2005; and may be regulated differently from those in flowering Osmont et al., 2007). Root system architecture manipulation plants (Hou et al., 2004). The bryophyte moss Physcomitrella is instrumental in the domestication and breeding of crop patens revealed a very ancient mechanism controlling the plants, because using water and nutrients from the soil in the development of tissues with a rooting function (Menand et al., most efficient manner affects a plant’s ability to survive in 2007). Physcomitrella possesses putative homologues of known stressful or poor soils. Changes in RSA can therefore have Arabidopsis LR regulators, many of which have no assigned huge impacts on the final yield of a crop (reviewed in de function (e.g. Axtell et al., 2007; Rensing et al., 2008). Dorlodot et al., 2007). Of the factors that control total RSA, Lateral root formation consists of four key stages: (i) LR formation and growth is one of the most important. stimulation and dedifferentiation of pericycle founder cells; Many of the hormonal and environmental signals affecting (ii) cell cycle re-entry and asymmetric cell divisions to give rise LR development also affect other components that have a to a lateral root primordium (LRP); (iii) LRP emergence bearing on RSA and the overall root surface area, namely, root through the outer layers of the PR via cell expansion; and (iv) New Phytologist (2008) 179: 595–614 www.newphytologist.org © The Authors (2008). Journal compilation © New Phytologist (2008) Tansley revie w Review 597 Fig. 2 Root anatomy. (a) Longitudinal section through an Arabidopsis primary root tip, showing the different cell types. LRC, lateral root cap (which is absent further up the root); Epi, epidermis (which is the outermost layer of the root above the root tip); Co, cortex; En, endodermis; P, pericycle; Vasc, vasculature (xylem and phloem); QC, quiescent centre (maintains the neighbouring stem cell population). (b) Transverse section through an Arabidopsis primary root. Epi, epidermis; RH, root hair; Co, cortex; En, endodermis; P, pericycle; XPP, xylem pole pericycle (the pericycle cells adjacent to the xylem tissue, from which lateral roots arise); Xy, xylem; Ph, phloem. In monocots, lateral roots arise from the phloem pole pericycle. Fig. 3 Aspects of auxin signalling during lateral root (LR) development. (a) A pulse of auxin (light grey) in the basal meristem (BM) primes a pericycle cell (dark grey) to become competent to form a lateral root initial cell. (b) Cells (white) leaving the basal meristem between cyclical auxin maxima are not specified to become LR initials. (c) The first primed pericycle cell arrives at a point where it can initiate LR development; meanwhile another pericycle cell (dark grey) is primed in the basal meristem by the subsequent auxin pulse. (d) Lateral root initiation begins with auxin-induced IAA14 degradation. This allows activation of the ARF7 and ARF19 transcription factors, which activate expression of LBD/ ASL genes. LBD/ASL proteins in turn activate cell cycle genes and cell patterning genes, enabling formation of a new lateral root primordium (LRP). Auxin also activates transcription of NAC1 to stimulate LR initiation, and at the same time induces expression of two ubiquitin ligases, CEGUENDO and SINAT5, which feed back to attenuate the auxin response.
Details
-
File Typepdf
-
Upload Time-
-
Content LanguagesEnglish
-
Upload UserAnonymous/Not logged-in
-
File Pages20 Page
-
File Size-