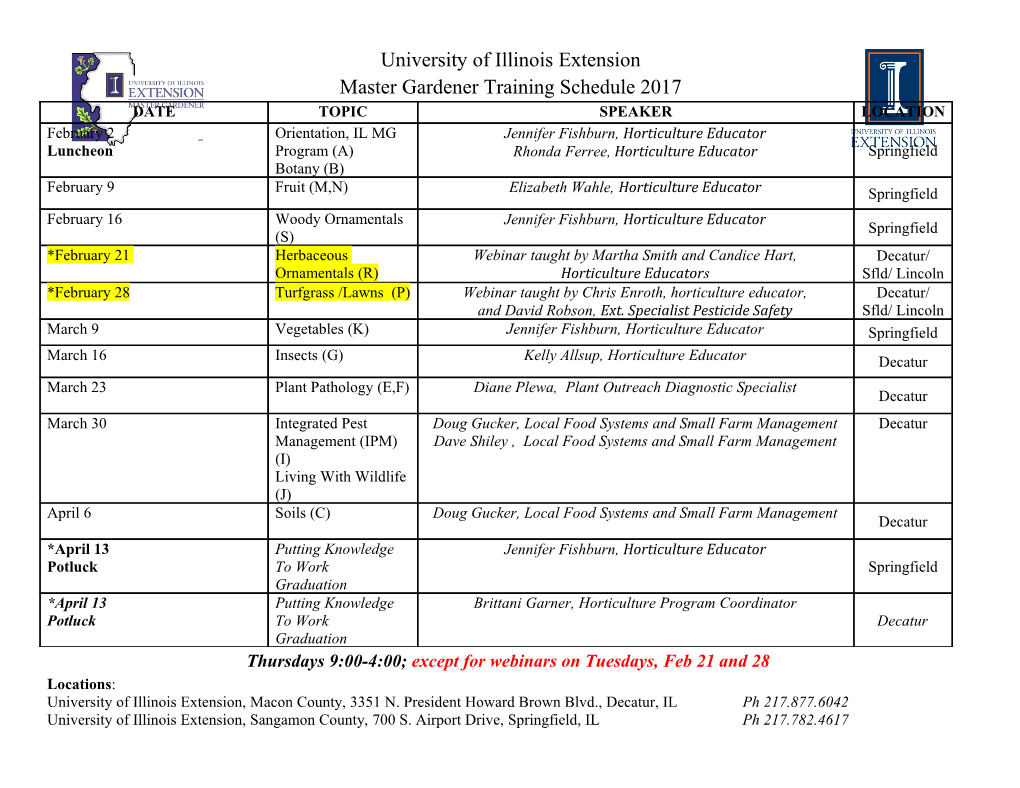
GAUGE THEORIES IN PARTICLE PHYSICS 1 QUARKS AND LEPTONS 1.1 The Standard Model The traditional goal of particle physics has been to identify what appear to be structureless units of matter and to understand the nature of the forces acting between them. Stated thus, the enterprise has a two-fold aspect: matter on the one hand, forces on the other. The expectation is that the smallest units of matter should interact in the simplest way; or that there is a deep connection between the basic units of matter and the basic forces. The joint matter/force nature of the enquiry is perfectly illustrated by Thomson’s discovery of the electron and Maxwell’s theory of the electromagnetic field, which together mark the birth of modern particle physics. The electron was recognized both as the ‘particle of electricity’—or as we might now say, as an elementary source of the electromagnetic field, with its motion constituting an electromagnetic current—and also as an important constituent of matter. In retrospect, the story of particle physics over the subsequent one hundred years or so has consisted in the discovery and study of two new (non-electromagnetic) forces—the weak and the strong forces—and in the search for ‘electron-figures’ to serve both as constituents of the new layers of matter which were uncovered (first nuclei, and then hadrons) and also as sources of the new force fields. This effort has recently culminated in decisive progress: the identification of a collection of matter units which are indeed analogous to the electron; and the highly convincing experimental verification of theories of the associated strong and weak force fields, which incorporate and generalize in a beautiful way the original electron/electromagnetic field relationship. These theories are collectively (and rather modestly, if uninformatively) called ‘the Standard Model’, to which this book is intended as an elementary introduction. In brief, the picture is as follows. There are two types of matter units: 1 ¯ leptons and quarks. Both have spin- 2 (in units of h) and are structureless at the smallest distances currently probed by the highest-energy accelerators. The leptons are direct generalizations of the electron, the term denoting particles which, if charged, interact both electromagnetically and weakly, and, if neutral, only weakly. The involvement of the electron in weak, as well as electromagnetic, interactions became clear quite early on, with the study of nuclear β-decay— though it took many years before it was conclusively established that the electron emitted in this process was invariably accompanied by a neutral leptonic 3 4 QUARKS AND LEPTONS partner, the antineutrino ν¯e. By contrast, the quarks—which are the fermionic constituents of hadrons, and thence of nuclei—are more like ‘strongly interacting analogues’ of the electron, since they interact via all three interactions, strong, electromagnetic and weak. This, indeed, is the basis for distinguishing between these two types of matter unit, a distinction which will presumably disappear if it eventually proves possible to unify all three types of force. At all events, the weak and electromagnetic interactions of both quarks and leptons are described in a (partially) unified way by the electroweak theory (see volume 2), which is a generalization of quantum electrodynamics or QED (chapters 8, 9 and 11); while the strong interactions of quarks are described by quantum chromodynamics or QCD (see volume 2), which is also analogous to QED. Both quarks and leptons act as sources of the electroweak field, while quarks alone act as the sources for the QCD field: we may speak of weak and strong ‘charges’ and ‘currents’ by analogy with similar concepts in QED. According to this picture, the observed ‘levels of structure’—atomic, nuclear and hadronic—are built up in a series of stages. Quarks interacting via QCD bind to form hadrons, such as neutrons, protons and pions. It has to be admitted at once that the details of this process are not yet fully understood, owing to the calculational difficulties of dealing with a strongly interacting quantum field theory. Residual interquark forces are then thought to give rise to the rather complicated observed forces between neutrons and protons, which are responsible for binding these nucleons to form nuclei. This stage is also not well understood, but is regarded as somewhat analogous to the way in which complicated interatomic forces are ultimately due to residual electromagnetic interactions between the constituents—for of course it is these latter interactions which bind electrons and nuclei into atoms. The reasons why, despite these gaps in the argument, we do nevertheless believe in QCD will be discussed in volume 2. As regards the weak force, it appears that, as its name already indicates, it is not strong enough to produce any binding effects among the systems studied so far. The reader will certainly have noticed that the most venerable force of all—gravity—is conspicuously absent from our story so far. Apart from a brief appearance in section 2.7, it will continue to be so. In practical terms this is quite reasonable, since its effect is very many orders of magnitude smaller than even the weak force, at least until the interparticle separation reaches distances far smaller than those we shall be discussing. Conceptually, also, gravity still seems to be somewhat distinct from the other forces which, as we have already indicated, are encouragingly similar. There are no particular fermionic sources carrying ‘gravity charges’: it seems that all matter gravitates. This of course was a motivation for Einstein’s geometrical approach to gravity. Despite remarkable recent developments in the theory of strings (Green et al 1987, Polchinski 1998), it is fair to say that the vision of the unification of all the forces, which possessed Einstein, is still some way from realization. To include any further discussion of these ideas would take us far beyond our stated aim. This book is not intended as a completely self-contained textbook on particle 1.2 LEVELS OF STRUCTURE: FROM ATOMS TO QUARKS 5 physics, which would survey the broad range of observed phenomena and outline the main steps by which the picture described here has come to be accepted. For this we must refer the reader to other sources (e.g. Perkins 2000, Martin and Shaw 1992). However, in the remainder of this chapter we shall offer a brief summary of the salient experimental facts which have established this current view of matter and forces, and in the next chapter an introduction to the conceptual framework needed to understand it. 1.2 Levels of structure: from atoms to quarks What kind of experiments are done to probe the structure of microscopically small systems? We may distinguish three basic types: scattering, spectroscopy and break-up experiments, the exploitation of which constitutes a common theme in the chain of discovery: atom → nucleus → hadron → quark. 1.2.1 Atoms → nucleus Around the turn of the 19th century, the experiments of Thomson and others established the charge and mass of electrons. In particular, the charge was exactly equal in magnitude to that of a singly ionized atom. Thus part, at least, of the atom had been ‘broken off’. Then, in 1909, Geiger and Marsden, of the University of Manchester in England, reported an unusual experimental finding. Working on a programme initiated by Rutherford, they were measuring how α particles are reflected by thin metal foils. In perhaps the understatement of the century, they remarked: It seems surprising that some of the α particles, as the experiment shows, can be turned within a layer of 6 × 10−5 cm of gold through an angle of 90◦, and even more. (Geiger and Marsden 1909) Many years later Rutherford, in a famous phrase, said that when he became aware of this result he was as amazed as if he had seen a shell bounce back from hitting a sheet of paper. It took him two years to find the explanation. The atom was known to be electrically neutral, and to contain negatively charged electrons of mass very much less than that of the atom. The question was: how is the heavy, positively charged matter distributed? It seems to have been generally thought of as being uniformly spread over the atomic volume, and a specific model of this type was proposed by J J Thomson in 1910. We shall call this the ‘soft’ model. In such a model, Rutherford (1911) noted, α particles are deflected very little in any single scattering process, so that large deflections can occur only as a result, as he put it, ‘of a multitude of small scatterings by the atoms of matter traversed’. However, ‘a simple calculation based on the theory of probability shows that the chance of an α particle being deflected through 90◦ is vanishingly small’. Rutherford went on: 6 QUARKS AND LEPTONS It seems reasonable to suppose that the deflection through a large angle is due to a single atomic encounter, for the chance of a second encounter of a kind to produce a large deflection must in most cases be exceedingly small. Asimple calculation shows that the atom must be a seat of an intense electric field in order to produce such a large deflection at a single encounter. Thus was the nuclear atom born, in which the atom contained a ‘hard’ constituent—the small, massive, positively charged nucleus. In more precise terms, according to the soft model the distribution of scattered particles falls off exponentially with the angle of deflection; the departure of the observed distribution from this exponential form is the signal for hard scattering. Geiger and Marsden found that about one in 20 000 α particles was turned through 90◦ or more in passing through a thin gold foil; by contrast a ‘soft’ model would predict a fraction of something like 1 in 103500! (Eisberg 1961, chapter 4).
Details
-
File Typepdf
-
Upload Time-
-
Content LanguagesEnglish
-
Upload UserAnonymous/Not logged-in
-
File Pages406 Page
-
File Size-