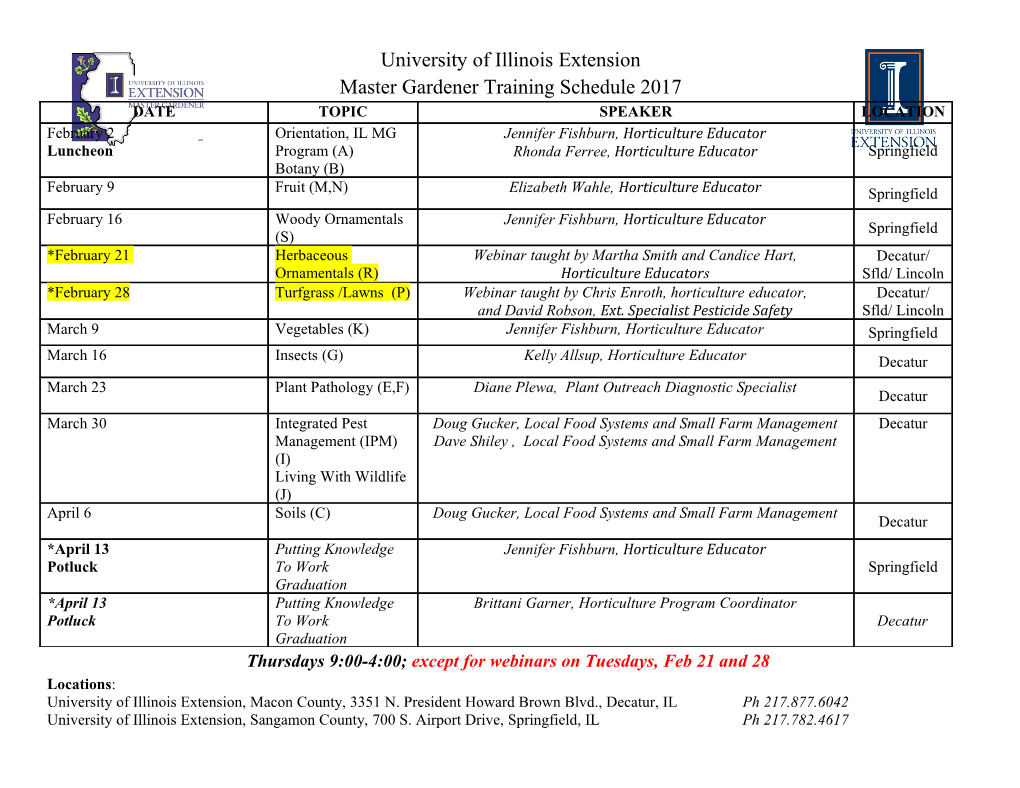
Lecture 2: Biopolymers • A biopolymer has a common backbone, a defined chemical start and a defined end. • The backbone of a biopolymer does not change with the sequence. • The bonding atom at the junction on the backbone must have at least 3 bonding options. • Carbon can exhibit properties of a metal and a non-metal. • Carbon can catenate – bond to itself and form long chains. • The Carbon-Carbon bond is stronger than the Carbon-Oxygen bond. • Silicon is much more abundant than carbon however the Silicon-Oxygen bond is much stronger than the Silicon-Silicon bond. • Carbon compounds are relatively inert or kinetically stable to hydrolysis and oxidation. • In general, organic reactions tend to be under kinetic control rather than thermodynamic control. • If something is thermodynamically controlled, it means that it is favorable in that direction. • Although many organic reactions are thermodynamically favourable (the product has less energy than the reactant), the rate of reaction is so slow as to be almost non-existent due to high activation energy. • This makes these reactions attractive for enzyme control. • The hydrogen molecule has a strong covalent bond and electrons are shared equally. • In an ionic bond, there is an unequal distribution of electrons due to unequal electron affinity between the atoms. • A polar covalent bond has dipole partial charges. • Hydrogen and carbon have similar electronegativity while oxygen has a higher electronegativity. • Major biopolymers: • The four main biopolymers are: fats, carbohydrates, protein and nucleic acid. • All linear biopolymers have a defined beginning and end. • Biopolymer synthesis is an anabolic process (requires energy input). • All biopolymers are synthesized in one direction only. • Some of the monomer is lost in polymerization, leaving a ‘residue’ incorporated in the growing chain. • Fats or lipids have the general formula (-CH2-)n. • Lipids are non-soluble due to a long hydrocarbon chain. • Carbohydrate or hydrated carbon has the general formula (H-C-OH) n. • Carbohydrates are extremely soluble with significant polarity. 2- • Ionic functional groups: COOH, NH3 and HPO4 • As the functional groups are increasingly oxidized they move from methyl groups to alcohol to aldehyde to ketone to carboxylic acid. • Aldehyde and ketone are carbonyl groups. + • The amine group is ionic, basic, has a pKa and is often positively charged at pH 7 (NH3 ). • The amide group is a derivative of carboxylic acid and is polar but non-ionic (NH2). • Information biopolymers contain a number of different monomers and the order of those monomers when they form a polymer is important and a template is required. The template must be copied faithfully. • Proteins are made up of 20 amino acids and differ in their side chains. • The amino acid side chains have very different chemical properties, unlike nucleic acid bases. • Proteins can be acidic, basic, polar or hydrophobic. • Two amino acids combine, by condensation polymerization to form a dipeptide. • There is an amide peptide bond and it has the properties of a secondary amide. • This reaction, translation, is extremely thermodynamically unfavourable due to the large amount of water around. • Hydrolysis is always favoured over condensation in an aqueous environment. The process in cells is done via a different reaction pathway. • The translation reaction occurs on ribosomes and water is excluded from the active site. • RNA catalyzes the reaction but ATP must activate amino acids first. • The peptide bond has 2 resonance structures, has a polarity so can form H bonds and has a partial double bond character so can’t rotate. • The peptide bond is able to be a proton donor and a protein acceptor. • Alpha amino acid: • All amino acids have the above general structure. • The alpha carbon is the carbon next to the functional group. • The R group hangs off the alpha carbon. • The carboxyl group at one end is negative while the amino group at the other is positive. • Hydrophobic amino acids all have a long side chain. • The only bonds in the side chain are C-C and C-H. • Both C and H have similar electronegativities and share the electrons fairly evenly with little or no dipole. • The longer the C-C and C-H chain, the more hydrophobic. • Aromatic amino acids have an aromatic ring in their side chain. • Proteins are detected by light absorbance at 280nm and an amino acid with an aromatic ring will absorb light. • Polar non-ionic amino acids have a side chain with a polarity, they will form hydrogen bonds but do not have a pKa i.e. do not become fully charged. • Carboxylic amino acids are negative at pH 7. • Basic amino acids are positive at pH 7. • Chirality happens if an atom has 4 different groups attached to it. • All amino acids are L isomers. • A D isomer placed into a cell will be a poison. Lecture 3: Charges on amino acids • To determine if it is an L or a D isomer, spell C-O-R-N using carbon, oxygen, the R group and nitrogen. • If CORN is spelt in the clockwise digestion it is an L isomer, if CORN is spelt in the anticlockwise direction it is a D isomer. • Charge is related to pH. • pH is the –log10 [H+] in moles/litre (M). • The lower the pH the higher the hydrogen ion concentration. • At pH 7, [H+] = [OH-]. • Acidic side chains - glutamate and asparate: • The acid is in equilibrium with its conjugate base. • When the concentration of H+ ions on the right hand side of the equation is increased, the equilibrium will shift to produce more of the acid and the acidic side chain (A-) is neutralised. • At low pH the acidic side chain becomes neutral, it has been protonated and is in the form COOH above. • Reacting the acid with sodium hydroxide introduces OH- ions which deprotonates the side chain and causes H+ to dissociate from the acid. • The H+ reacts with OH- to form water. • The OH- ions have a stronger affinity for the H + and cause it to dissociate from the AH. • At high pH, the acidic side chain becomes negative. • At low pHs, the acidic side chain is neutral. • At high pHs, the acidic side chain is negative. • Basic side chains – arginine, lysine & histidine: • The basic group is charged when it is protonated. • When the basic group is deprotonated it has no charge. • When H+ ions are added to the right hand side of the equation, they will react with the base to form a positive side chain. • At low pH the basic side chain becomes positive. • When sodium hydroxide is added, the OH- ions react with H+ ions to form water and the BH+ will denature to form the base (B). • At high pH the basic side chain become neutral. • Glycine at pH 1: • The pKa is the pH at the midpoint of dissociation-association. • Glycine at the pKa of the carboxyl group, pH 2.2: • The higher the pH, the more the equilibrium will shift towards the right, i.e. the protonated form. • For each 1-pH increase, the number that is in the base form will increase by a factor of 10. • At pH 7, almost all is in the basic form. • At pH 9.6, the pKa of the amino group has been reached: • Half is protonated; the other half has lost a proton. • At pH 12, the majority is in the base form minus a proton. • The pI is the pH where the charge on the amino acid is exactly zero, it is the average of the highest and the lowest pKa. • The pI for glycine above will be the average of 2.2 and 9.6. • If the amino acid is placed in an electric field at this point it will not move. • If you place the amino acid in a solution at a higher pH it will be negative (due to the COO-). + • If the solution has a lower pH the amino acid will be positive (due to the NH3 ). • A buffer is a solution that resists a change in pH if you challenge it with an acid or a base. • A solution is a buffer +/- 1 pH unit either side of its pKa. • A basic side chain will raise the pI, i.e. the pH at which it is neutral is also raised. • The molecule will be increasingly positive below the pI and increasingly negative above the pI. • An acidic side chain will lower the pI. • Charges on proteins: • The formation of the peptide bond neutralises the carboxyl and amino group charges on the alpha carbon but not the charges on the side chains. • Not all side chains have a charge – only lys, arg, his, glu and asp. • The charge on each side chain is amino acid dependent. • There is always one N terminal and one C terminal in the backbone of a protein. • Different proteins have different native charges and the overall charge on a protein will depend on the sequence and the pH. • Determining the pI of a protein: • It can be predicted from the difference between the sum of the acidic side chains (asp + glu) and the sum of the basic side chains (lys + arg + his). • It is determined experimentally by techniques such as isoelectric focusing. • The protein is placed in a pH gradient and subjected to an electric field. • The protein moves towards its pI. • Those proteins with more acidic residues will have a lower pI. • Those proteins with more basic residues will have a higher pI. • At pH lower than the pI of the protein, the protein will be positive. • At pH higher than the pI of the protein, the protein will be negative. • For example, at pH 7 a protein with a pI of 9 will be positive while a protein with a pI of 5 will be negative.
Details
-
File Typepdf
-
Upload Time-
-
Content LanguagesEnglish
-
Upload UserAnonymous/Not logged-in
-
File Pages14 Page
-
File Size-