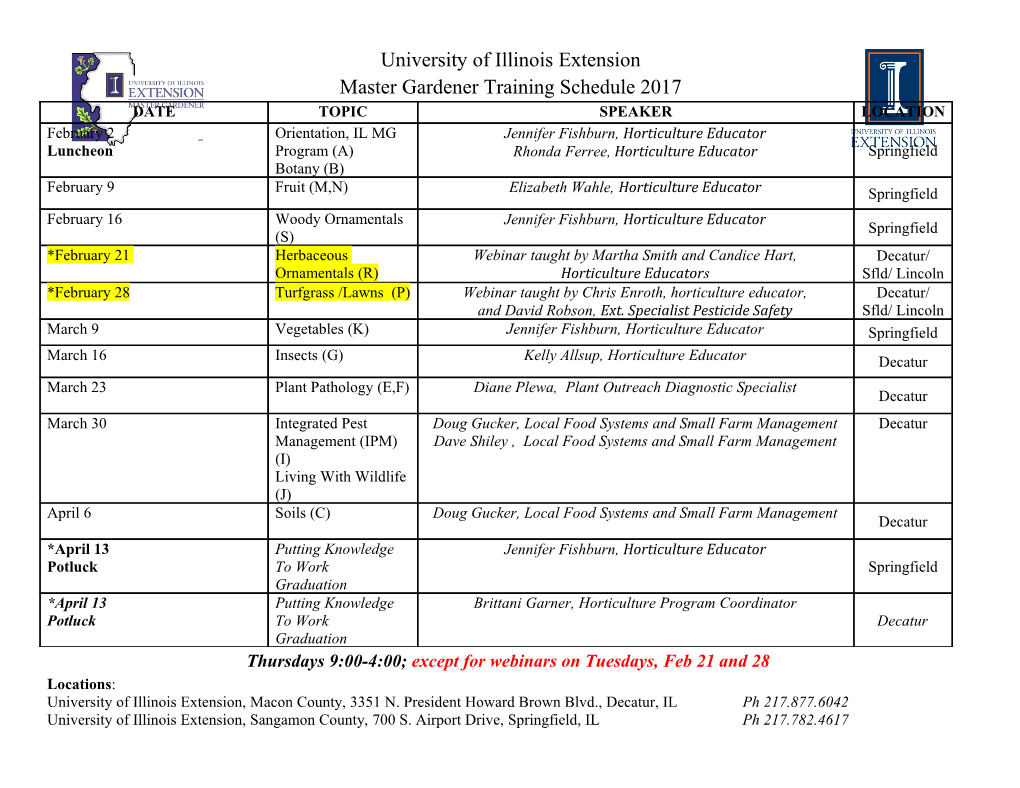
Phase separation dynamics in colloid-polymer mixtures: the effect of interaction range Isla Zhang and Paul Bartlett School of Chemistry, University of Bristol, Bristol, BS8 1TS, UK. C. Patrick Royall Wills Physics Laboratory, University of Bristol, Tyndall Avenue, Bristol BS8 1TL, UK, School of Chemistry, University of Bristol, Bristol BS8 1TS, UK, Centre for Nanoscience and Quantum Information Tyndall Avenue, Bristol BS8 1FD, UK. Malcolm A. Faers Bayer CropScience AG, 40789, Monheim am Rhein, Germany. Colloid-polymer mixtures may undergo either fluid-fluid phase separation or gelation. This depends on the depth of the quench (polymer concentration) and polymer-colloid size ratio. We present a real-space study of dynamics in phase separating colloid-polymer mixtures with medium- to long-range attractions (polymer- colloid size ratio qR = 0:45 − 0:89), with the aim of understanding the mechanism of gelation as the range of the attraction is changed. In contrast to previous studies of short-range attractive systems, where gelation occurs shortly after crossing the equilibrium phase boundary, we find a substantial region of fluid-fluid phase separation. On deeper quenches the system undergoes a continuous crossover to gel formation. We identify two regimes, ‘classical’ phase separation, where single particle relaxation is faster than the dynamics of phase separation, and ‘viscoelastic’ phase separation, where demixing is slowed down appreciably due to slow dynamics in the colloid-rich phase. Particles at the surface of the strands of the network exhibit significantly greater mobility than those buried inside the gel strand which presents a method for coarsening. I. INTRODUCTION (higher polymer concentration) may also raise fc-r. During phase separation, if fc-r reaches volume fractions at which Dynamical arrest in soft matter can generally be divided dynamical arrest occurs (fc-r ∼ 0:57−0:59), demixing is sup- into two classes, glasses and gels. Arrest is exhibited by a pressed and a gel results [4, 14, 15]. In the absence of crys- wide variety of systems, including those which can be treated tallisation, such a system is nonetheless metastable to col- as spheres with attractions which include colloid-polymer loidal liquid-gas coexistence. Fig. 1 shows a schematic sketch mixtures [1] and protein solutions [2, 3]. Dynamical arrest oc- of the effect of interaction range on the phase behaviour (ig- curs upon either a global increase in density (vitrification) or noring crystal phases) [9, 16]. arrested phase separation (gelation). In the latter case, phase Although such polydisperse dense (colloidal) liquids may separation is suppressed as the system undergoes arrest to be metastable to the formation of size-segregated crystals form a non-equilibrium gel state, which we define as a per- [17], to the best of our knowledge this has not been observed colating assembly of particles [4, 5] which do not relax on the in experiments. Consequently, while most theoretical treat- experimental timescale. ments necessarily consider the equilibrium monodisperse case In colloid-polymer mixtures, addition of sufficient polymer where colloidal liquids are found up to fc-r . 0:5 (a regime causes demixing into two or more phases [6, 7]. In a one- in which dynamical arrest is not expected), the local vol- component description, the degrees of freedom of the poly- ume fraction in the ‘arms’ of a gel may be higher in experi- mers are formally integrated out, leading to an effective at- ments due to polydispersity. Although mean-field theories ex- traction between the colloidal particles whose range is fixed ist where the metastable gas-liquid binodal and spinodal can be calculated [18], such predictions have limited accuracy for by the ratio of the polymer and colloid diameters qR [8] and whose strength is set by the polymer concentration. To a rea- many polymer-colloid size ratios [11]. sonable approximation, polymer concentration plays the role Previous work has largely focused on gelation in systems arXiv:1209.3973v1 [cond-mat.soft] 18 Sep 2012 of inverse temperature. The topology of the phase diagram is with short-range attractions, typically with polymer-colloid well-known: short-range interactions lead to only fluid-crystal size ratios qR . 0:2[4, 19–23] such that there is no equilib- phase coexistence, along with a metastable fluid-fluid binodal. rium colloidal liquid phase. In such systems, gelation occurs However as the interaction range increases to qR ≈ 0:3, fluid- upon crossing the metastable fluid-fluid spinodal[4, 5, 21, 23]. fluid phase separation produces a stable colloidal liquid and In contrast, phase separation and its arrest in colloid-polymer colloidal gas [1, 9, 10]. mixtures with medium-to-long range attractions, where there While the topology of the phase diagram is well-known, is a stable colloidal liquid phase, are much less understood. the case of metastable amorphous states presents some chal- Recent simulation[24] and experimental data[25, 26] suggest lenges to theory and simulation [11]. Smaller polymers lead to that a liquid-like colloid-rich phase exists, which grows con- tinuously in length scale as the system ages. a shorter interaction range qR, so that upon demixing, the col- loids are closer together and thus the volume fraction of the For long-range (qR & 0:3) systems, we suggest two regimes colloid-rich phase fc-r is increased [12, 13]. Deep quenches of interest in relation to the gas-liquid binodal - shallow and 2 deep quenches. For shallow quenches, the kinetics of phase attractive range increases, the region where phase separation separation in colloid-polymer mixtures is analogous to liquid- is uninterrupted by gelation expands. In addition, the mech- liquid demixing in molecular systems [27]. The structural anism of domain growth is studied in detail. We find that evolution can be described by the Cahn-Hilliard theory of upon quenching, coarsening occurs predominately through spinodal decomposition [28, 29]. Several regimes are dis- enhanced particle mobility at the surface of the strands of the tinguished by the growth rate of the wavelength of density gel network. fluctuations. Immediately after quenching, a fastest-growing dominant mode qm appears with a wavelength lm, which ex- hibits time-dependent growth, with the power-law exponent II. MATERIALS AND METHODS changing from 1=3 to 1 as the system evolves from the diffu- sive into the hydrodynamic coarsening regime. The charac- A. Colloid-polymer mixture I: Emulsions + HEC teristic domain size associated with the wavelength grows un- til it approaches the capillary length l = (g=Drg)1=2, where c Our first model system consisted of a low polydispersity g is the interfacial tension and Dr the density difference be- emulsion of poly(dimethyl siloxane) (Silicone DC200 fluid tween the two phases, at which point any gravity-driven flow 10cSt, Serva) dispersed in a refractive index-matched mixed may rupture the bicontinuous structure [30]. Previous work solvent of 1,2-ethane diol (EG) and water. The emulsion on spinodal decomposition in colloid-polymer mixtures has was stabilized against aggregation by a combination of three shown that the crossover between diffusive and hydrodynamic commercial non-ionic surfactants (w/w ratio 1:0.417:0.015); regimes is broad and continuous, and both theory and experi- tristyrylphenol ethoxylate, 16EO (Tanatex), PEO-PPO-PEO, ment indicate that, depending on the importance of hydrody- M 4950, 30% PEO (Pluronic P103, BASF) and to give a namic interactions, the exponent may vary between 0.2 and w small negative charge, the anionic surfactant sodium bis(2- 1.1 [27, 31]. ethyl 1-hexyl) sulfosuccinate (Aerosol OT, Cytec). Small Quenching more deeply, the volume fraction of the col- droplets (PDMS:surfactant 1:0.12) had a hydrodynamic ra- loidal liquid increases [9, 18]. The volume fraction of the dius s=2 = 142 nm and a size polydispersity of 0.10, while colloid-rich phase may then approach f ∼ 0:58, the point at large droplets (PDMS:surfactant 1:0.05) had a hydrodynamic which slow dynamics set in for hard spheres. Coarsening may radius s=2 = 277 nm and a size polydispersity of 0.18. The therefore be suppressed and the bicontinuous network gener- index-matched continuous phase had a viscosity of 5.42 mPa s ated by the initial spinodal decomposition becomes partially ± 0.02 mPa s, as measured by capillary viscometry, a refrac- or, in the limit of very short-range systems, even completely tive index of 1.396 at 543 nm, and a density of r = 1:067 arrested on the experimental timescale [15]. The viscosity dif- c g cm−3± 0.005 g cm−3. The density of the PDMS oil is ference between the phases may be reminiscent of the vis- lower than the continuous phase (r = 0:934 g cm−3 ± 0:006 coelastic phase separation proposed by Tanaka [15]. m g cm−3) so that the dispersions cream in a gravitational field. The density mismatch of the oil is Dr = rc − rm = 0:13 ± 0.01 g cm−3. To screen out long-range electrostatic interac- tions and generate hard-sphere-like interactions between the emulsion drops we add 3 mM potassium chloride, which gives a Debye length of 6 nm. A dye, Sudan Black, was also added to increase contrast between colloid-rich and colloid- poor phases such that the phase separation could be identified by eye. Sudan Black preferentially adsorbs to the surface of the PDMS droplets, and so the colloid-rich phase appears dark and the colloid-poor phase light. FIG. 1 Schematic phase diagram showing the effect of attractive Hydroxyethylcellulose (HEC), which is a water-soluble range on the gas-liquid coexistence line and the intersection between non-ionic cellulose derivative, was used as the depletant. Two the coexistence line and the glassy region.
Details
-
File Typepdf
-
Upload Time-
-
Content LanguagesEnglish
-
Upload UserAnonymous/Not logged-in
-
File Pages8 Page
-
File Size-