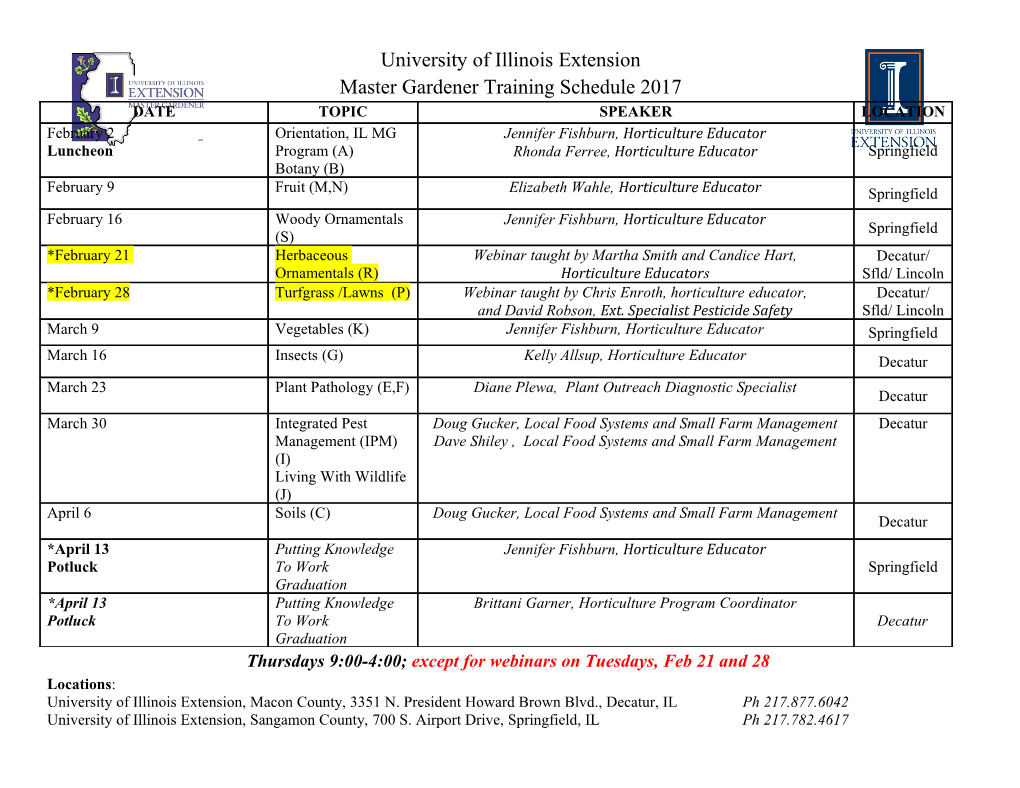
Rev 2, 4 April 1999 CHAPTER 2: PLASMA CONFINEMENT AND TRANSPORT TABLE OF CONTENTS 1. INTRODUCTION ............................................................................1 2. MECHANISMS OF TRANSPORT IN A TOKAMAK ..................................5 3. EXPERIMENTAL DESCRIPTION OF CONFINEMENT AND TRANSPORT..... 18 3.1. Introduction ......................................................................18 3.2. General Results for Ohmic and L-Mode ......................................19 3.3. Regimes with Edge Transport Barrier (H-mode) and Recommended Regime for ITER.......................................................................... 22 3.4. Regimes with Internal Transport Barrier .....................................25 3.4.1. Barriers associated with reversed or weak magnetic shear ......25 3.4.2. Other improved core confinement regimes without edge radiation .........................................................................28 3.5. Enhanced Confinement with Edge Radiation.................................... 31 4. L-H AND H-L TRANSITION PHYSICS .................................................35 4.1. Physical Processes of Transition ..............................................36 4.2. Edge Pedestal ....................................................................38 4.3. Power Threshold Scaling....................................................... 41 5. IMPACT OF GLOBAL INSTABILITIES ON TRANSPORT ..........................48 5.1. Sawteeth ..........................................................................49 5.2. Edge Localized Modes ..........................................................52 6. GLOBAL ENERGY CONFINEMENT SCALINGS..................................... 57 6.1. Introduction ......................................................................57 6.2. H-Mode Global Confinement Database....................................... 59 6.3. Power Law Scaling Expressions ..............................................61 6.3.1. ELMy H-mode thermal confinement scaling...................... 63 6.3.2. ELM-free H-mode thermal confinement scaling.................. 66 6.3.3. L-mode thermal confinement scaling ..............................67 6.4. Point and Interval Estimation for the Confinement Time in ITER .........71 7. SCALING STUDIES WITH SIMILAR DIMENSIONLESS PHYSICS PARAMETERS.......................................................................... 76 7.1. Basics .............................................................................76 7.2. Discharges with Identical Dimensionless Parameters .......................80 7.3. Results of Experiments with Similar Dimensionless Parameters ..........81 7.4. Limitations on Transport Scaling Studies with Similar Dimensionless Parameters........................................................................ 85 7.5. Summary .........................................................................88 IPB-Chapter 2 i Confinement & Transport Expert Group Confinement Database & Modeling Expert Group Rev 2, 4 April 1999 8. 1-D TRANSPORT MODELS................................................................ 90 8.1. Introduction ......................................................................90 8.2. Theoretical and Semi-Empirical Transport Models ..........................92 8.3. Plasma Profile Database ........................................................97 8.4. Results of 1-D Modeling Tests ................................................99 8.5. Predictions of Transport Models for ITER.. .................................105 9. PARTICLE TRANSPORT: HYDROGEN, HELIUM, IMPURITIES .................110 10. MOMENTUM CONFINEMENT.......................................................... 117 11. SUMMARY ..................................................................................123 Appendix: ITER ENERGY CONFINEMENT PROJECTION .............................127 A1. Point and Interval Estimation based on Log-Linear and Log Non-Linear Scaling Expressions ..........................................................127 A2. Framework for Interval Estimation .........................................138 REFERENCES ....................................................................................144 LIST OF TABLES ................................................................................166 LIST OF FIGURES ..............................................................................166 IPB-Chapter 2 ii Confinement & Transport Expert Group Confinement Database & Modeling Expert Group Rev 2, 4 April 1999 1. INTRODUCTION The most developed concept for realizing continuous energy (electricity) production from fusion reactions is the tokamak, based on an axisymmetric toroidal plasma. In recent years there has been considerable development of databases and accumulation of knowledge of the behavior of tokamak plasmas around the world, and these make it possible to design an experimental fusion reactor for energy production. However, a degree of uncertainty still exists in predicting the confinement properties and plasma performance in such a device. A precise theory of the classical collisional transport losses has been developed. Since this does not completely explain the transport processes across magnetic surfaces (as discussed in Section 2), additional processes driven by plasma turbulence are required to be taken into account. Significant theoretical efforts are being devoted to understanding the cross-field transport in tokamaks due to the turbulence and a few models are broadly consistent with present experiments. On the other hand, since tokamaks with a range of sizes, operating parameters and heating powers have been constructed, empirical scaling laws derived from these are useful for predicting plasma performance in any new device. Furthermore, the empirical scalings serve as a benchmark for theoretical models. One expects that predictions with such scaling laws will be improved if one imposes dimensional constraints on the form of these laws in the scaling studies. It is also recognized that transport codes solving radial transport equations numerically are also useful for obtaining quantitative predictions. As a result, three approaches are being pursued at the moment for providing predictions for confinement: these are (a) derivation of empirical global scaling laws, (b) non-dimensionally similar studies, and (c) one dimensional transport modeling codes. The main strengths of the empirical energy confinement scaling method (described in Section 6) are its simplicity and the fact that all of the physical processes are contained within the data. Its main weakness is that the modeling of the energy confinement time τE by a simple log-linear form, or even by more sophisticated forms, can only, at best, be a very approximate description of the physical processes taking place, since no knowledge of the heating, temperature or density profiles, or atomic physics for that matter, is built into the analysis. The IPB-Chapter 2 1 Confinement & Transport Expert Group Confinement Database & Modeling Expert Group Rev 2, 4 April 1999 log-linear form itself is equivalent to assuming that a single turbulence mechanism with one scale size is responsible for the transport. This seems unlikely to be the case for the ELMy H-mode, the operational mode foreseen for ITER, where the core region may be dominated by short wavelength turbulence of the gyroBohm type, and the behavior in the edge region is possibly determined by MHD events such as the ELMs (Edge Localized Modes). These two processes will scale differently with the main scaling parameter ρ*, the normalized Larmor radius (≡ ρi/a). There is also the possibility that there are hidden parameters that are not being taken into account in the analysis. One parameter which may affect the confinement in some situations, but which is not presently being taken into account, is the edge neutral pressure; another possible important parameter is the Mach number of the toroidal rotation M (≡ Vφ/cs). Both these parameters are currently being added to the database, but their effect on the ITER predictions is as yet unclear. The degradation of confinement associated with proximity to operational limits, such as the Greenwald density limit, β limit and H-mode power threshold, is still an area of active studies and not yet well quantified by the available database. One can assess the statistical uncertainty in the ITER energy confinement time τITER using the techniques that are described in Section 6.5. The 95% interval estimate for the prediction of τITER from the ELMy H-mode log-linear scaling Eq. (26) is τITER = (4.4–6.8) s. With this narrow uncertainty interval, ITER would definitely ignite, although operation at higher current or higher density might be required to preserve ignition at the lower end of this interval. A larger interval, τITER = (3.5–8.0) s, has been derived by taking various non log- linear forms and other considerations into account. At the lower end of this range the minimum Q (≡ thermonuclear power/input power) would be above 6. The dimensionless physics parameter scaling technique (described in Section 7) overcomes one of the shortcomings of the energy confinement scaling approach, namely the influence of the plasma profiles on the confinement. In fact, in the simple case of a single dominant turbulence mechanism, one can obtain directly, by a simple projection, from those of current experiments, the temperature and density profiles in ITER provided the source profiles of heat and particles are the same. A
Details
-
File Typepdf
-
Upload Time-
-
Content LanguagesEnglish
-
Upload UserAnonymous/Not logged-in
-
File Pages164 Page
-
File Size-