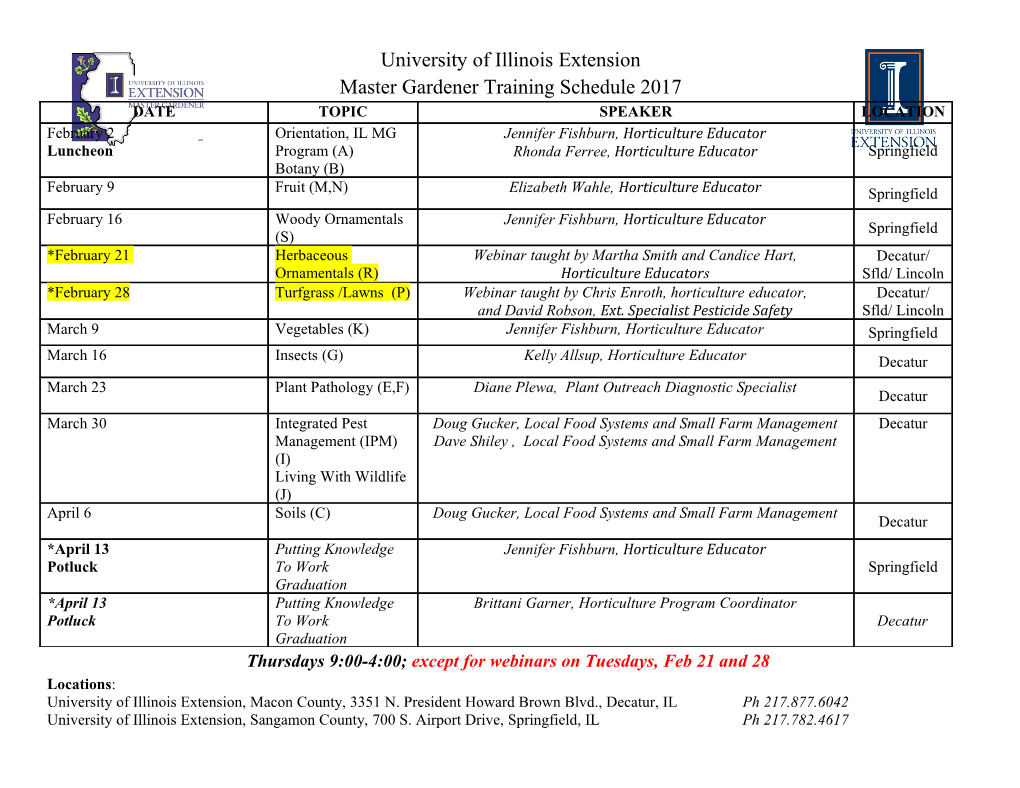
DISSERTATION / DOCTORAL THESIS Titel der Dissertation /Title of the Doctoral Thesis „Single Phonon Quantum Optics - An experimental exploration of a silicon photonics quantum memory“ verfasst von / submitted by Ralf Riedinger, M.Sc. angestrebter akademischer Grad / in partial fulfilment of the requirements for the degree of Doktor der Naturwissenschaften (Dr. rer. nat.) Wien, 2018 / Vienna 2018 Studienkennzahl lt. Studienblatt / A 796 605 411 degree programme code as it appears on the student record sheet: Dissertationsgebiet lt. Studienblatt / Physik field of study as it appears on the student record sheet: Betreut von / Supervisor: Univ.-Prof. Dr. Markus Aspelmeyer Abstract Acoustic phonons, the energy eigenstates of mechanical vibrations, possess a plethora of interesting features for quantum information processing. For example, they can serve as compact quantum memories with long life times, or can transduce quantum states between a variety of otherwise incompatible quantum systems. Particularly interesting among those are infrared photons in the telecommunication wavelength band, due to their widespread use in quantum communication. Using artificial optical and mechanical resonances, they can interact with phonons by radiation pressure. However, controlling individual excitations of motion in micromechanical resonators has thus far been restricted to the domain of microwave radiation, while optical control remained an outstanding goal. In this thesis, I describe how this can be achieved experimentally, employing quantum optics protocols. First, the operation of silicon optomechanical crystals in the quantum regime is demonstrated by creating non-classical photon-phonon pairs through optome- chanical down conversion. This quantum interface is subsequently used to characterize heralded single phonons, and to generate quantum entanglement between two remote mechanical oscillators. The realization of these classic quantum optics experiments with single phonons establishes mechanical quantum memories in silicon photonics as a useful resource for future quantum networks. Zusammenfassung Akustische Phononen, die energetischen Eigenzust¨andevon mechanischen Vibrationen, besitzen eine Vielzahl an interessanten Eigenschaften f¨urdie Verarbeitung von Quanten- information. Beispielsweise k¨onnensie als langlebige und kompakte Quanteninformation- sspeicher eingesetzt werden, oder Quantenzust¨andezwischen anderweitig inkompatiblen Systemen ¨ubertragen. Aufgrund ihrer technischen Relevanz sind hierbei Photonen im optischen Telekommunikations-Frequenzband von besonderem Interesse. Mit Hilfe von k¨unstlich erzeugten optischen und akustischen Resonanzen, k¨onnendie Photonen mit Phononen mittels Strahlungsdruck interagieren. Jedoch war die Kontrolle einzelner Be- wegungsanregungen in mikromechanischen Resonatoren bisher auf den Mikrowellenbere- ich beschr¨ankt,wohingegen das Ziel einer Manipulation durch Laser unerreicht blieb. In dieser Dissertation beschreibe ich, wie dies experimentell mithilfe von quantenoptischen Protokollen erreicht werden kann. Zun¨achst wird gezeigt, dass optomechanische Kristalle im Quantenbereich betrieben werden k¨onnen,indem nichtklassische Photon-Phonon Paare mittels optomechanischer parametrischer Fluoreszenz erzeugt werden. Diese Quanten- schnittstelle wird daraufhin verwendet um einzelne Phononen zu charakterisieren, sowie Quantenverschr¨ankungzwischen zwei entfernten mechanischen Oszillatoren zu erzeugen. Die Demonstration dieser klassischen Quantenoptik Experimente mit einzelnen Phononen zeigt, dass mechanische Quanteninformationsspeicher eine vielversprechende Ressource f¨urk¨unftigeQuantennetzwerke darstellen. Contents Abstract I Contents III 1. Introduction1 2. Theory of Cavity Optomechanics5 2.1. The Optomechanical Interaction . .7 2.2. Characterization of the Components . 21 2.3. Single Phonon Quantum Optics . 31 2.4. Basic Protocols . 46 3. Physical realization 57 3.1. Photonic Crystal Cavities . 57 3.2. Phononic Crystal Resonators . 59 4. Non-classical correlations between single photons and phonons from a me- chanical oscillator 61 4.1. Abstract . 65 4.2. Main Text . 66 References . 72 4.3. Supplementary Information . 76 5. Hanbury Brown and Twiss interferometry of single phonons from an op- tomechanical resonator 87 5.1. Abstract . 91 5.2. Main Text . 91 References . 98 5.3. Supplementary Information . 102 6. Remote quantum entanglement between two micromechanical oscillators 111 6.1. Abstract . 117 6.2. Main Text . 118 References . 125 6.3. Supplementary Information . 129 7. Outlook 139 Bibliography 141 Appendices 169 III Contents A. Notations 171 B. Theory 173 B.1. The Thermal Mechanical State . 173 B.2. Emitted Mode Envelope in a Quasi-Continuously Driven System . 174 B.3. The Cross-Correlation in an Optomechanical System . 177 B.4. Generation and Verification of Remote Mechanical Entanglement . 182 C. Curriculum Vitae 185 Acknowledgments 187 IV 1. Introduction Quantum optics is a field of research investigating and exploiting the consequences of the quantization of the electromagnetic field and its interaction with matter. Triggered by the invention of the laser, it experienced major advances, shaping our understanding of the foundations of physics and enabling new technologies for the manipulation of quan- tum systems. In this thesis, I employ quantum optical techniques to control individual excitations of motion, called phonons, in micromechanical oscillators. The experiments reported here demonstrate that optomechanical systems, in particular optomechanical crystals, can be a valuable resource for future optical quantum information architectures. They can serve as a quantum memory on an integrated silicon photonics platform, directly interfaced with photons in the conventional optical telecommunication wavelength band. Quantum Optics Early quantum optics experiments, such as the observation of particle-like features of light emitted by atoms [Cla74; KDM77], demonstrated the applicability of quantum field theory for optical experiments. Further, these non-classical emitters allowed to study quantum entanglement [FC72; AGR81], a counter-intuitive consequence of applying the superposition principle to correlations of particles [EPR35; Bel64]. Conversely, it was realized that light could be utilized to manipulate the internal [Kas50] and external degrees of freedom of atoms [Ash70]. Later on, the quantum optical control techniques were refined and extended to non-linear optical processes in bulk crystals [BW70; HM86] and various atom-like systems, such as defects in solid state materials [Gru+97] or superconducting qubits [Cla+88; NPT99; Wal+04a]. The ability to precisely manipulate these systems led to various applications, such as precision metrology, quantum simulation, quantum computation and quantum communication. Of particular interest is the coherent control of individual excitations in these systems. They can form the quantum analogue to a single bit of information, called qubit, which is the basis for the majority of quantum information protocols. Cavity Optomechanics Optical manipulation of massive objects has been demonstrated early on [Bet36; Ash70], however, it was limited to motion in the classical domain. Quantum control over motion was later on achieved in atomic systems [Die+89; Mee+96] using laser cooling techniques. In recent years, efforts to extend this quantum control to the motion of massive objects has attracted considerable attention [AKM14]. Relying on artificial instead of atomic resonances, this field of research was named cavity optomechanics. Technological advances in micromechanics and optics are promising to enable quantum control over massive, artificial degrees of freedom. On the one hand, this allows for an investigation on the 1 1. Introduction validity of quantum theory for macroscopic systems, some of them even visible to the naked eye [Tho+08]. On the other hand, the mechanical element can act as transducer [Rab+10] and memory [CG04; Fio+11; Cha+11b; Saf+11] in various quantum information applications. As the optical and mechanical resonances are artificially created, they can be designed to work at almost arbitrary frequencies. For example, a single mechanical element coupled to two electromagnetic resonances in vastly different frequency domains can act as bidirectional transducer between them [And+14; Vai+16]. Furthermore, the freedom of design can also be used to make mechanical quantum memories available for wavelength ranges with notoriously few usable atomic resonances. One such example is the conventional optical telecommunication band (C-band) around a wavelength of 1550 nanometers, which is of enormous interest for long distance quantum communication protocols, due to the high transmission of photons in this range in optical fibers. To expand quantum communication beyond the typical absorption length of these fibers, quantum repeaters have been proposed [Bri+98; Dua+01], which require mem- ory elements interfaced to the traveling infrared photons. Particularly interesting is the possibility to have an array of optomechanical devices with closely spaced resonances in the C-band. This allows for wavelength division multiplexing of quantum communication channels, thereby achieving very high operational bandwidths. The individual mechan- ical elements can at the same time maintain long memory times, exceeding the current limitations of other broadband telecom quantum memory techniques, like rare earth ion doped crystals [Lau+10; Bus+14; Sag+15]. However,
Details
-
File Typepdf
-
Upload Time-
-
Content LanguagesEnglish
-
Upload UserAnonymous/Not logged-in
-
File Pages195 Page
-
File Size-