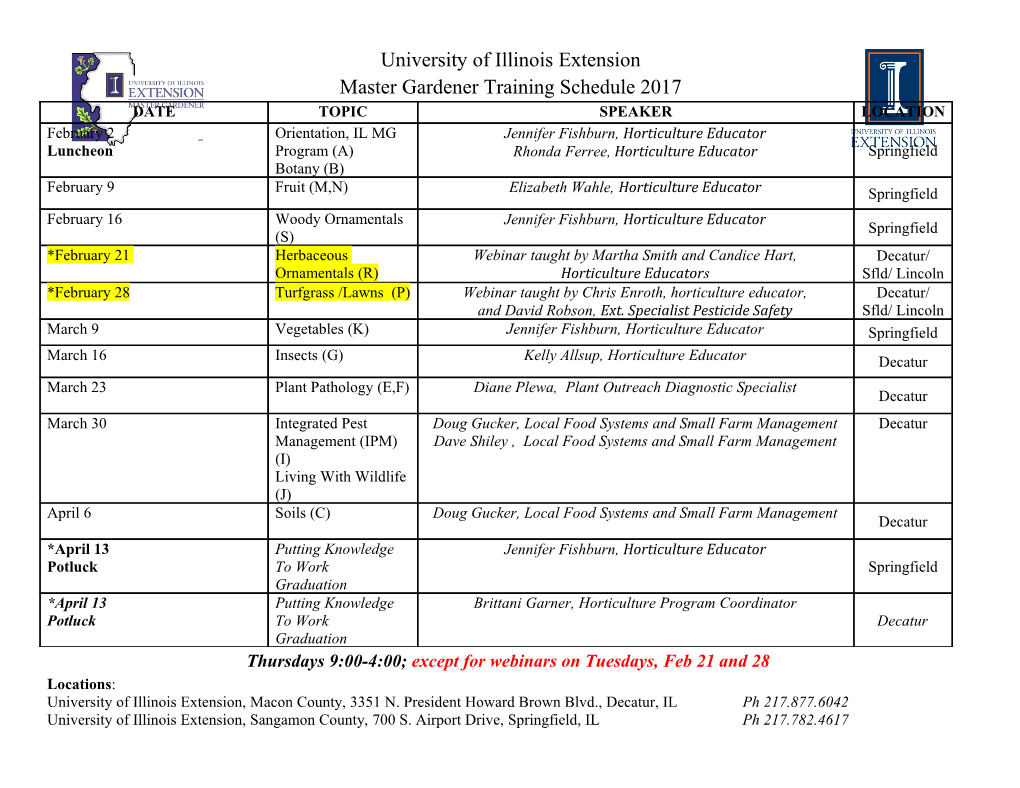
JP0050803 JAERI-Cont" 2000-006 6 5 • Vacuum Ultraviolet Ar Excimer Emission Initiated by High Intensity Laser Produced Electrons Shoichi Kubodera and Wataru Sasaki Department of Electrical and Electronic Engineering and Photon Science Center, Miyazaki University Gakuen Kibanadai Nishi 1-1, Miyazaki, 889-2192 Japan We have observed Ar2* emission using a tabletop femtosecond high intensity laser as an excitation source. High intensity laser produced electrons via an optical field induced ionization (OFI) process initiated the Ar2* production kinetics, which made themselves analogous to those produced in an electron beam produced plasma. A fast conductive cooling of the OFI plasma was found to be appropriate to initiate the excimer formation kinetics more efficiently. Keywords: Vacuum ultraviolet, Excimer molecule, Short pulse laser, Conductive cooling There have been considerable demands for the development of compact short wavelength lasers in the vacuum ultraviolet (VUV) spectral region. Such compact short wavelength lasers would be applicable to various scientific and industrial fields, such as photochemistry, biological science, and new types of materials processing. Currently available practical compact VUV lasers are the ArF excimer laser at 193 tun and the F2 laser at 157 run, both of which are excited by a compact discharge device. Recently more attention is paid to short wavelength lasers in the VUV in the future optical lithography industry. Rare gas excimers have long been one of the very few laser media in the VUV spectral region [1]. The emission wavelength of Ar2* is 126 run which is long enough to use transmission optical elements such as MgF2 and LiF. The Kr2* laser has an even longer emission wavelength centered at 147 mn which relaxes the conditions for optics and would become a competitor to the F2 laser at 157 run. Rare gases have a contrast to chemically active fluorine in the F2 laser. A recent rapid progress of the ultrashort pulse high intensity laser technology makes it possible to control major plasma parameters in an optical field induced ionization (OFI) plasma [2]. We have pointed out that this OFI plasma may simulate certain plasma parameters of an electron beam produced plasma, where rare gas excimer production kinetics are optimized for laser oscillation. The temperature of an electron beam produced plasma could be simulated by choosing appropriate high intensity laser parameters such as a focused intensity, wavelength, and the degree of the polarization. The electron density found in an electron beam produced plasma may also be reproduced by producing a desired charged state of a plasma by an adequate choice of such laser parameters. High pressure operation in the electron beam excitation for efficient excimer production may be reproduced in a vacuum chamber by using a pulsed gas jet to provide a temporally and spatially localized medium. The use of a pulsed gas jet will minimize unfavorable nonlinear effects such as beam focusing and defocusing caused by high intensity laser propagation and will maximize a three body recombination process for the efficient rare gas excimer production [1]. In order to obtain a large excitation length, a plasma channeling or the use of a hollow optical fiber may be utilized although this has not been verified yet. In this paper, we report on the demonstration of the Ar2* production using a tabletop femtosecond high intensity laser as an excitation source. High intensity laser produced electrons via the OFI process initiated the Ar2* production kinetics, which made themselves analogous to those produced in the electron beam produced plasma. A fast cooling of the OFI plasma was found to be appropriate to initiate the excimer formation kinetics more efficiently. 285 - JAERI-Conf 2000-006 A tabletop Ti:sapphire oscillator amplifier system produced a femtosecond high intensity pulse at 800 nm. Maximum output energy was 2 mJ in a pulse width of 130 fs at a repetition rate of 10 Hz. The laser pulse was focused into a rare gas jet placed in a vacuum chamber by using a thin lens with a focal length of 35 cm. A maximum focused laser intensity in vacuum was measured to be 5 x 1014 Wcm"2, which was high enough to tunnel ionize Ar to singly ionized Ar ion [3]. A pulsed gas jet was synchronized to the laser operation at 10 Hz by a pulse generator. Laboratory grade Ar was used as rare gases and their maximum backing pressures were 35 atm, which was limited by a capacity of our vacuum system. A X/4 waveplate was inserted in a laser axis to control the degree of the polarization of the laser beam. VUV emission from the interaction region was detected by a visible photomultiplier tube with a sodium salicylate scintillator coupled to a 0.2-m VUV spectrometer. Typical spectral resolution was 0.5 nm. An optical axis of a VUV spectrometer was placed either parallel to or orthogonal to a short pulse laser irradiation axis in a vacuum chamber to observe VUV emissions. When it was placed onto the laser irradiation axis, a Ceratron detector was used instead of a photomultiplier to minimize the detection of the intense 800 nm laser light. Time-resolved signals of the VUV emissions were recorded using a 2 GHz digital oscilloscope. Time-integrated spectral information was acquired using a boxcar integrator. Both electronic devices were connected to a personal computer for data storage and further data processing. Fig. 1 shows time-integrated spectra observed using an Ar gas jet. In both spectra, the laser beam was linearly polarized and its focused intensity was 5 x 1014 Wcm"2. A backing pressure of Ar was 20 atm. 2 (a) CO Ar2* 1 = Ar* (3D6 - 4s ) 1 Ar* (3p6 - 4s) 1 110 120 130 140 150 Wavelength (nm) 15th (2nd order) Ar* 7th 13th (b) (2nd order) 11th 1 (2nd order) I 1 - L II Ar * f 2 s J w 1 100 110 120 130 140 150 Wavelength (nm) Fig. 1 Emission spectra from an Ar gas jet observed orthogonal to the laser irradiation axis (a), and onto the laser axis (b). - 286 - JAERI-Conf 2000-006 Fig. l(a) shows a spectrum observed when a spectrometer was placed such as to observe the VUV emission orthogonally to the high intensity laser irradiation axis. We have observed Ar2* emission centered at 126 nm and two Ar* resonance lines at 105 and 107 run. No other emissions including ionic lines were observed in the spectral region between 30 nm and 300 nm. Ar* acts as a precursor of Ar2* in the three body recombination process. When the spectrometer was placed onto the high intensity laser axis, several high order harmonic signals were observed as well as Ar2* and Ar* emissions as shown in Fig. l(b). This spectrum has not been corrected for the spectral response of the Ceratron that had a rapid decrease of its sensitivity toward a longer wavelength in this spectral region. Referring to the intensity ratio between Ar2* and Ar* found in Fig. l(a), the Ar2* emission intensity would become twice as large as that of the 7th order harmonic observed in Fig. l(b). Making an estimate with solid angle correction based on an emission geometry, Ar2* intensity becomes at least several tens of times as large as that of the 7th order harmonic, indicating the difference of the pulse duration of both emissions which was beyond the time resolution of this measurement. Note that no excimer emissions were observed when a self breakdown plasma was produced by a nanosecond Q-switched Nd:YAG laser at an intensity of 10" Won"2. A nanosecond laser produced plasma was uncontrollably heated and its density also uncontrollably increased, that did not fulfill the conditions of the excimer production. Fig. 2 shows the intensities of Ar2* emission and the 5th order harmonic signal as a function of the degree of polarization of the laser beam. A circularly polarized laser beam emerged at 0° and 90°, and linear polarization occurred at 45°. The 5th order harmonic signal becomes its maximum and minimum when the laser beam was linearly and circularly polarized, respectively, as the nonlinear optics predicts. On the other hand, the Ar2* emission intensity has almost no dependence on the degree of the polarization. The electron temperature of the OFI plasma should be very sensitive to the polarization of an incoming laser beam [2]. Calculated average electron temperatures of our Ar plasma are 4 eV and 70 eV for linearly and circularly polarized laser beams, respectively. Such a large difference should have been reflected in the excimer production kinetics. The resulting emission intensities, however, have no difference, indicating a rapid cooling of the laser produced electrons in a plasma. A cooling mechanism of an OFI Ar plasma has been evaluated numerically based on the energy transport of electrons [4,5]. Neglecting the hydrodynamic motion and density gradients of such a plasma in a subpicosecond time scale, the plasma cooling is considered due to thermal conduction of electrons after \ 1 1 1 1 \ 1 1 8 X X •e CO 1 ° ft # # 5 1 - "8 LU V f < o 1 1 1 1 1 135 20 40 60 80 Scale of Waveplate (deg) Fig. 2 Emission intensities of Ar2* and the 5th order harmonic as a function of the degree of the polarization of the laser beam. Scales at 0° and 90° correspond to the circularly polarized beam, and that at 45° represents a linearly polarized beam. 287 - JAERI-Conf 2000-006 the plasma initiating laser pulse. For a plasma with strong temperature gradients or in the collisionless regime, electrons are assumed to be free-stream rather than diffuse.
Details
-
File Typepdf
-
Upload Time-
-
Content LanguagesEnglish
-
Upload UserAnonymous/Not logged-in
-
File Pages4 Page
-
File Size-