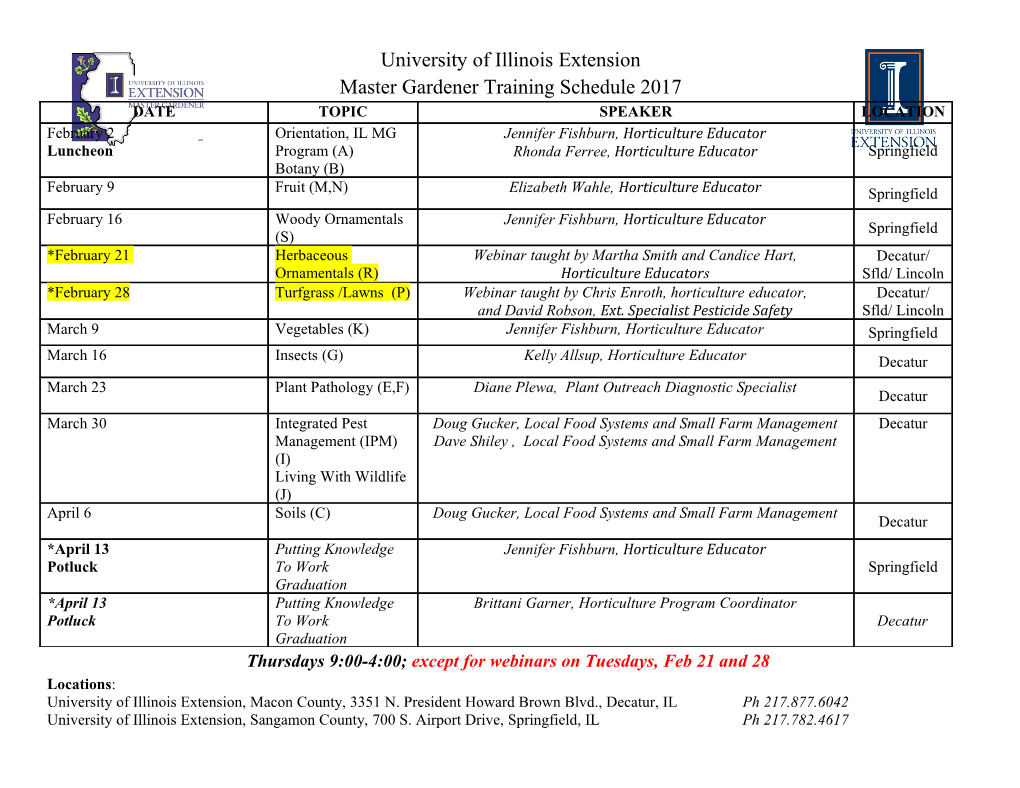
Redox potential of the terminal quinone electron acceptor QB in photosystem II reveals the mechanism of electron transfer regulation Yuki Kato1, Ryo Nagao, and Takumi Noguchi1 Division of Material Science, Graduate School of Science, Nagoya University, Nagoya 464-8602, Japan Edited by Pierre A. Joliot, Institut de Biologie Physico-Chimique, Paris, France, and approved December 4, 2015 (received for review October 12, 2015) Photosystem II (PSII) extracts electrons from water at a Mn4CaO5 (Em values). Both forward and backward electron transfers are cluster using light energy and then transfers them to two plas- important; backward electron transfers control charge recombina- toquinones, the primary quinone electron acceptor QA and the tion in PSII, and this serves as photoprotection for PSII proteins secondary quinone electron acceptor QB. This forward electron (5, 14–17). PSII involves specific mechanisms to regulate for- transfer is an essential process in light energy conversion. Mean- ward and backward electron transfer reactions in response to while, backward electron transfer is also significant in photopro- environmental changes. For instance, in strong light, some spe- tection of PSII proteins. Modulation of the redox potential (Em) gap cies of cyanobacteria increase the Em of Pheo to facilitate charge of QA and QB mainly regulates the forward and backward electron recombination. Specifically, they exchange D1 subunits origi- transfers in PSII. However, the full scheme of electron transfer nating from different psbA genes to change the hydrogen bond regulation remains unresolved due to the unknown Em value of interactions of Pheo (16–20). On the other hand, it was found QB. Here, for the first time (to our knowledge), the Em value of QB that impairment of the Mn4CaO5 cluster led to a significant in- reduction was measured directly using spectroelectrochemistry in crease in the Em of QA by ∼150 mV (21–27). This potential in- combination with light-induced Fourier transform infrared differ- crease was thought to inhibit forward electron transfer to QB to − − ence spectroscopy. The Em(QB /QB) was determined to be approx- promote direct relaxation of QA without forming triplet-state imately +90 mV and was virtually unaffected by depletion of the Chl, a precursor of harmful singlet oxygen (2, 5, 14, 15, 17, 23). BIOPHYSICS AND Mn CaO cluster. This insensitivity of E (Q −/Q ), in combination − + 4 5 m B B In addition, charge recombination of QA with P680 prevents COMPUTATIONAL BIOLOGY − + with the known large upshift of Em(QA /QA), explains the mecha- oxidative damage by high-potential P680 (5). However, the full nism of PSII photoprotection with an impaired Mn4CaO5 cluster, in mechanism of photoprotection by the regulation of the quinone which a large decrease in the Em gap between QA and QB pro- electron acceptor E values remains to be resolved, because the − m motes rapid charge recombination via QA . Em of QB has not been determined conclusively, and the effect of Mn4CaO5 cluster inactivation on it has not been examined (5). photosynthesis | spectroelectrochemistry | FTIR Although the Em of the single reduction of QB has been es- timated to be ∼80 mV higher than that of QA from kinetic and n oxygenic photosynthesis in plants and cyanobacteria, photo- thermodynamic data (28–32), so far no reports have measured Isystem II (PSII) has an important function in light-driven water the Em of QB directly. In contrast, the Em of QA was measured oxidation, a process that leads to the generation of electrons and extensively using chemical or electrochemical titrations and de- protons for CO reduction and ATP synthesis, respectively (1–3). termined to be approximately −100 mV for oxygen-evolving PSII 2 – Photosynthetic water oxidation also produces molecular oxygen (21 27). The main reason for this difference is due to the fact that the QA reaction can be monitored readily by fluorescence mea- as a byproduct, which is the source of atmospheric oxygen and − sustains virtually all life on Earth. PSII reactions are initiated by surement in that an increase in fluorescence indicates QA formation light-induced charge separation between a chlorophyll (Chl) di- mer (P680) and a pheophytin (Pheo) electron acceptor, leading Significance + − to the formation of a P680 Pheo radical pair (4, 5). An electron + hole on P680 is transferred to a Mn4CaO5 cluster, the catalytic In photosynthesis, photosystem II (PSII) has a function of ab- center of water oxidation, via the redox-active tyrosine, YZ (D1- stracting electrons from water using light energy and trans- Tyr161). At the Mn4CaO5 cluster, water oxidation proceeds ferring them to a quinone molecule. In addition to the forward through a cycle of five intermediates denoted Sn states (n = 0–4) electron transfer in PSII, which is essential in energy conversion, (6, 7). On the electron acceptor side, the electron is transferred backward electron transfer is important in photoprotection of − PSII proteins. Forward and backward electron transfers in PSII are from Pheo to the primary quinone electron acceptor QA and regulated by the redox potential (E ) gap of quinone electron then to the secondary quinone electron acceptor QB (8, 9). QA m acceptors, Q and Q . However, the regulation mechanism is still and QB have many similarities: they consist of plastoquinone A B (PQ), are located symmetrically around a nonheme iron center, unclear because Em of QB has not been determined. We directly and interact with D2 and D1 proteins, respectively, in a similar measured Em of QB using an electrochemical method in combi- manner (Fig. 1) (10, 11). However, they play significantly dif- nation with Fourier transform infrared spectroscopy. Our results ferent roles in PSII (8, 9). Q is only singly reduced to transfer an clearly explain the mechanism of electron transfer regulation in A PSII relevant to photoprotection. electron to QB, whereas QB accepts one or two electrons. When − Q is doubly reduced, the resultant Q 2 takes up two protons to B B Author contributions: Y.K. and T.N. designed research; Y.K. and R.N. performed research; form plastoquinol (PQH2), which is then released into thylakoid Y.K. and T.N. analyzed data; and Y.K. and T.N. wrote the paper. membranes. Differences between QA and QB could be caused by The authors declare no conflict of interest. differences in the molecular interactions of PQ with surrounding This article is a PNAS Direct Submission. proteins in QA and QB pockets, although the detailed mechanism 1To whom correspondence may be addressed. Email: [email protected] remains to be clarified (12, 13). or [email protected]. Electron transfer reactions in PSII are highly regulated by the This article contains supporting information online at www.pnas.org/lookup/suppl/doi:10. spatial localization of redox components and their redox potentials 1073/pnas.1520211113/-/DCSupplemental. www.pnas.org/cgi/doi/10.1073/pnas.1520211113 PNAS Early Edition | 1of6 Downloaded by guest on October 2, 2021 In this study, we directly measured the Em of QB in PSII using spectroelectrochemistry and light-induced FTIR difference spectroscopy. The effect of Mn4CaO5 cluster depletion on the Em value was also examined. Spectroelectrochemistry has been used to accurately measure the Em values of cofactors in various redox proteins (47, 48) including redox cofactors in PSII (24, 25, 49, 50). FTIR spectroelectrochemistry, which has the additional merit of being able to provide structural information, has also been used to investigate redox reactions of biomolecules and proteins (48, 50–54). This method was recently applied to the nonheme iron center of PSII to examine the effect of Mn de- pletion on the Em value and obtain structural information around the nonheme iron (50). The results in our study showed − that the Em of the first reduction of QB [Em(QB /QB)] was much higher than previously estimated, and the Em of the second re- − duction [Em(PQH2/QB )] was higher than the first reduction. Furthermore, we showed that Mn depletion hardly affected the Em values of QB, in contrast to the large change in the Em of QA (21–27). With these results, the mechanism of photoprotection of PSII when the Mn4CaO5 cluster is inactivated is now clearly explained. Results FTIR difference spectra of PSII core complexes from a ther- mophilic cyanobacterium Thermosynechococcus elongatus upon illumination of a single saturating flash were measured at a series of electrode potentials ranging from +250 to +50 mV (Fig. 2A). At the highest potential of +250 mV, a prominent peak was − observed at 1,480 cm 1, which is typical of the CO/CC stretching − vibration of a QB semiquinone anion (46), whereas at the lowest potential of +50 mV, a similar peak was found at a slightly lower −1 − frequency of 1,478 cm , which is typical of QA (55, 56). A significant change was observed in the ester C=O region (1,750– − − 1,700 cm 1): the intensity of a positive peak at 1,745 cm 1 de- − creased gradually, and a peak at 1,721 cm 1 increased, as the potential was lowered from +250 to +50 mV. The former and − latter peaks were shown previously to belong to QB /QB and − QA /QA differences, respectively, and were proposed to be good markers for discriminating between QB and QA reactions (46). Each peak was assigned to the 132-ester C=O vibration of the nearby Pheo (PheoD2 and PheoD1 for QB and QA, respectively; Fig. 1), which is electrostatically and/or structurally coupled to QB(A) and hence affected by its photoreduction (46). The fre- Fig. 1. Redox cofactors in PSII and the electron transfer pathway (blue ar- −1 rows). For the PSII structure, the X-ray crystallographic structure at 1.9-Å quency difference between 1,745 and 1,721 cm is attributed to resolution (Protein Data Bank ID code 3ARC) (9) was used.
Details
-
File Typepdf
-
Upload Time-
-
Content LanguagesEnglish
-
Upload UserAnonymous/Not logged-in
-
File Pages6 Page
-
File Size-