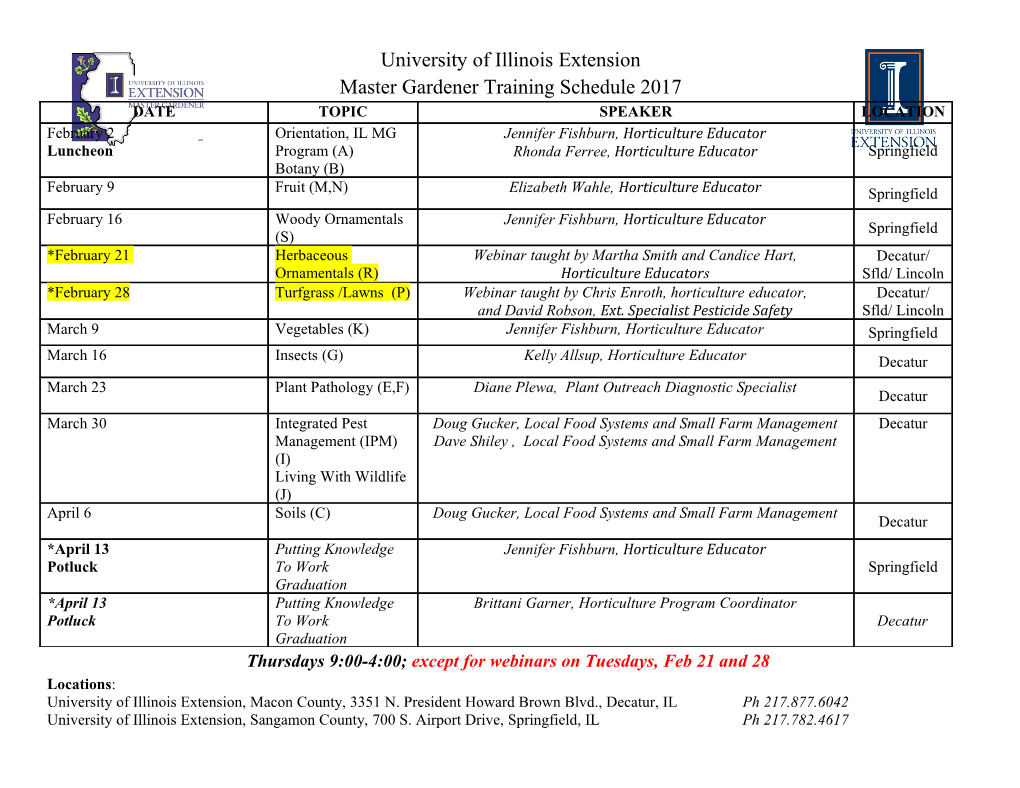
PHYSICAL REVIEW ACCELERATORS AND BEAMS 20, 072401 (2017) Permanent magnet based dipole magnets for next generation light sources Takahiro Watanabe,1,2,* Tsutomu Taniuchi,1 Shiro Takano,1,2 Tsuyoshi Aoki,1 and Kenji Fukami1,2 1Japan Synchrotron Radiation Research Institute (JASRI), 1-1-1 Kouto, Sayo, Hyogo 679-5198, Japan 2RIKEN SPring-8 Center (RSC), 1-1-1 Kouto, Sayo, Hyogo 679-5148, Japan (Received 26 January 2017; published 31 July 2017) We have developed permanent magnet based dipole magnets for the next generation light sources. Permanent magnets are advantageous over electromagnets in that they consume less power, are physically more compact, and there is a less risk of power supply failure. However, experience with electromagnets and permanent magnets in the field of accelerators shows that there are still challenges to replacing main magnets of accelerators for light sources with permanent magnets. These include the adjustability of the magnetic field, the temperature dependence of permanent magnets, and the issue of demagnetization. In this paper, we present a design for magnets for future light sources, supported by experimental and numerical results. DOI: 10.1103/PhysRevAccelBeams.20.072401 I. INTRODUCTION At the SPring-8 third generation synchrotron radiation After the considerable successes of third-generation facility in Japan, a major upgrade (known as SPring-8-II) ring-based light sources and the inauguration of linear- has been discussed that would target substantial improve- accelerator-based X-ray Free Electron Lasers (XFEL) [1,2], ments in light source performance [6]. For that purpose, a one of the main streams for future light sources is to five-bend achromat lattice is being designed at an electron develop a ring-based light source that can produce orders- energy of 6 GeV [12]. In the new lattice, four out of the five of-magnitude higher brilliance than that generated by third bending magnets are LGBs, and the remaining one at the generation light sources. With that goal in mind, newly center of the unit cell is a normal bending magnet (NB). designed storage rings for future light sources are based on The resulting natural emittance of the bare lattice is estimated to be down around 150 pm · rad, and is expected multibend lattices with more than two bending magnets in 100 each unit cell [3–9]. In addition, most of those projects do to be reduced further to around pm · rad by additional not rely merely on the increase in the number of bending damping at the insertion devices. In addition to this low magnets to obtain smaller emittance [10]; various dipole emittance, another underlying concept for the project is magnets with field gradients have been proposed and have energy saving, both for achieving significantly better light played key roles in pushing the limit. A bending magnet source performance and for reducing power consumption. with a transverse field gradient, which is equivalent to an Another essential concept is related to stability and reli- off-axis quadrupole magnet, is expected to lower the ability. Because one of the strengths of a ring-based light emittance, taking advantage of its compactness and the source is its stability and reliability, we deem it important to availability of damping partition control. A bending magnet maintain those strengths in the next generation light source. with a longitudinal field gradient, which we refer to These concepts are taken into consideration throughout the design of SPring-8-II. hereinafter as a longitudinal gradient bend (LGB) is also This is where permanent magnets come into play. expected as a new option to facilitate low emittance lattices Permanent magnets consume no energy in user operations [11]. In an LGB, electrons are bent by a large angle when a [13], and there is no risk of power supply failure unless dispersion function is small and vice versa, thereby electricity has to be supplied for some specific reason. suppressing quantum excitation in electron bunches. These features match the requirements for future accel- Thus, the development of bending magnets with field erators, wherein stability and reliability are taken for gradients is one of the key issues for future accelerators. granted. The absence of winding coils could also be beneficial if a new lattice is to be designed to have a high *[email protected] packing factor. The physical interference with an adjacent magnet, a beam position monitor and/or other components Published by the American Physical Society under the terms of could be relaxed. the Creative Commons Attribution 4.0 International license. Further distribution of this work must maintain attribution to However, we suggest that there are practical challenges the author(s) and the published article’s title, journal citation, to using permanent magnets as the main magnets (e.g., and DOI. dipole, quadrupole, and sextupole magnets) in future light 2469-9888=17=20(7)=072401(9) 072401-1 Published by the American Physical Society WATANABE, TANIUCHI, TAKANO, AOKI, and FUKAMI PHYS. REV. ACCEL. BEAMS 20, 072401 (2017) sources. Our claim is supported by the fact that no modern A. Field adjustability light sources use permanent magnet based main magnets. One of the key challenges with a permanent magnet is First, there is no straightforward way to readily tune the how to adjust its magnetic field. The main magnets of magnetic field, especially over a wide dynamic range. accelerators are often required to be tunable in precise and Second, the magnetic field generated by a permanent repeatable ways, for which electromagnets have played magnet is known to be temperature dependent [14,15], important roles. However, adjustable fields have been which affects the electron energy and lattice functions when proposed and discussed for permanent magnets [24–31], the ambient temperature changes. Third, demagnetization especially for providing variable focusing fields. of permanent magnets in undulators have been observed at Although not every case requires the magnetic field to be – several light sources [16 20], which should be avoided for adjusted dynamically over a wide range, some amount of the main magnets. Further, it is necessary to discuss the adjustability is required either to tune the magnetic fields feasibility of producing the newly proposed bending precisely at the outset or to compensate for demagnetiza- magnets with field gradients as permanent magnet based tion later on. For our purposes, we have developed the main magnets for light sources. adjustable-field dipole magnet that is illustrated in Fig. 1. In this paper, we discuss and summarize the issues that In nonadjustable magnets, the magnetic circuit is should be addressed in the design of permanent magnet designed so that as much as possible of the magnetic flux based main magnets for future light sources, and we generated by the permanent magnet goes into a closed loop propose possible solutions. The proposed designs are tested through the beam axis [32]. In Fig. 1, a portion of the and verified numerically and experimentally. magnetic flux is intentionally leaked out of the closed loop Although our main work is targeted mainly at develop- by making other loops (dashed line). Thus, it becomes ments for SPring-8-II [21–23], this paper presents a general possible to change the magnetic flux density on the beam discussion on the possibilities of using permanent magnets axis by mechanically moving the outer plates: for future light sources and other accelerators. Other multipole magnets could be designed on a permanent ϕ ϕ − ϕ − ϕ ðrÞ magnet basis as well, but we here focus on the develop- B ¼ gap ¼ pm loss op : ð Þ gap S S 1 ments in dipole magnets. gap gap B ϕ S II. DESIGN AND PERFORMANCE Here gap, gap, and gap are respectively the magnetic flux OF PERMANENT MAGNET-BASED density, the magnetic flux, and the cross sectional area in ϕ DIPOLE MAGNETS the gap. The magnetic flux, pm, is generated by permanent magnets. A difference between an electric circuit and a The main specifications for the SPring-8-II bending ϕ magnetic circuit is that the loss of flux, loss, may not be magnets are summarized in Table I. In an NB, the dipole negligible mainly because of the finite permeability of iron. magnetic field is homogeneous so that electrons experience Now, the part of the remaining flux, ϕ , that is intention- a constant dipole field along the magnet except for edge op ally leaked to the outer plates can be described as a function fields. In the LGBs specified in Table I, the dipole field of the outer plate position, r ¼ðx; y; zÞ. The flux ϕ can be comes in a stepwise distribution with three different op strengths, so electrons are bent with increasing or decreas- ing kick angles in a single magnet. In the following, we address and discuss the main issues regarding the practical production of permanent magnet based dipole magnets. TABLE I. Specifications of bending magnets for SPring-8-II (interim). Magnet NBa LGBb Maximum field (T) 0.95 0.79 Effective length (m) 0.42 1.75 Gap (mm) 25 25 GFRc (mm) Æ6 Æ6 Magnets per cell 1 4 FIG. 1. A magnetic circuit for an adjustable-field C-shaped Magnets per ring 44 176 dipole magnet. The red solid curve represents a closed loop of the aNormal bending magnet. magnetic flux for the beam axis. The red dashed curve is for leaked bLongitudinal gradient bending magnet. flux. The arrows show easy axes of permanent magnet pieces. The cGood field region where field deviation is within 0.1%. green squares are spaces for Fe-Ni alloys (see Sec. II B). 072401-2 PERMANENT MAGNET BASED DIPOLE … PHYS. REV. ACCEL. BEAMS 20, 072401 (2017) estimated by numerical simulations, but in the Appendix Hitachi Metals, Ltd.) and iron.
Details
-
File Typepdf
-
Upload Time-
-
Content LanguagesEnglish
-
Upload UserAnonymous/Not logged-in
-
File Pages9 Page
-
File Size-