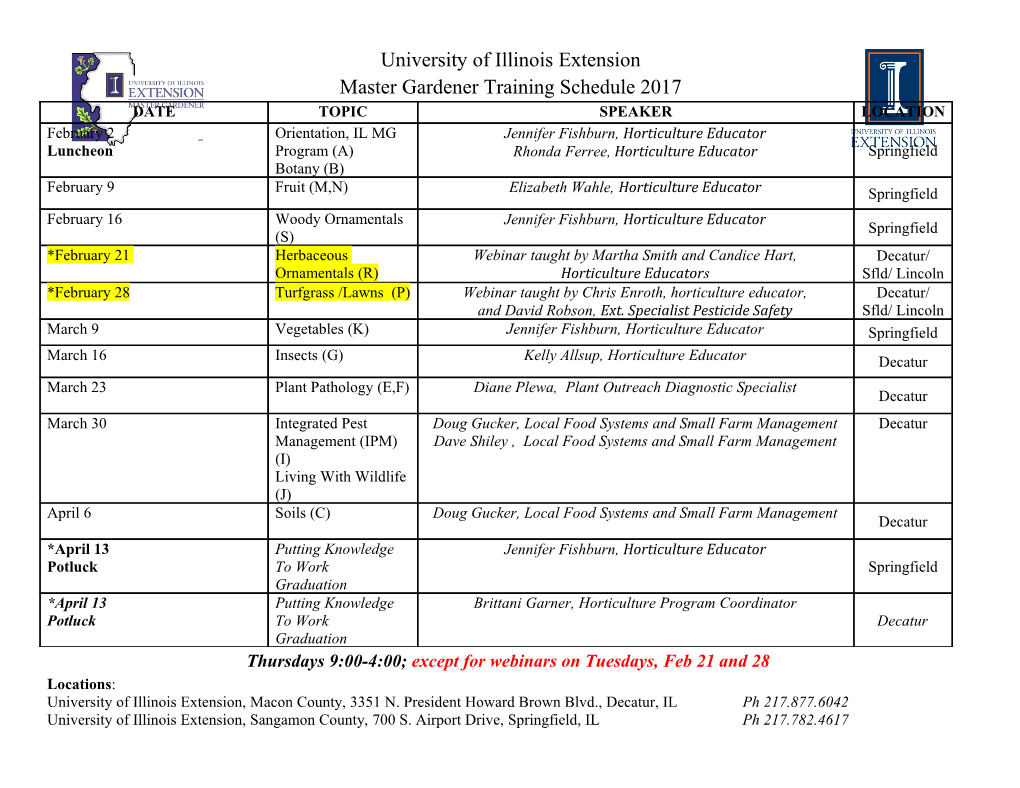
GEANT4 simulations of the muenster dual phase xenon tpc GEANT4-Simulationen zur Munsteraner¨ 2-Phasen xenon-tpc Bachelorarbeit Lutz Alth¨user Westf¨alische Wilhelms-Universit¨atM¨unster Institut f¨urKernphysik AG Prof. Dr. C. Weinheimer Themensteller: Prof. Dr. C. Weinheimer Zweitgutachter: Prof. Dr. J. Wessels M¨unster,September 2015 Contents iii Contents 1 Introduction 1 2 The Muenster dual phase xenon time projection chamber 3 2.1 Detector geometry and functionality . 3 2.2 Light collection efficiency and light yield . 5 2.2.1 Calibration sources and positioning . 7 2.2.2 Gamma interactions . 8 3 Monte-Carlo simulations of the Muenster time projection chamber 11 3.1 GEANT4 . 11 3.1.1 Detector geometry . 12 3.1.2 Particle tracking and basic detector response . 14 3.2 Scintillation light propagation . 16 3.2.1 Light collection efficiency . 16 3.2.2 Light yield . 21 3.3 Calibration simulations of the Muenster time projection chamber . 25 3.3.1 57Co..................................... 26 3.3.2 60Co..................................... 29 3.3.3 137Cs..................................... 33 3.3.4 83mKr.................................... 37 4 Conclusion and outlook 41 Bibliography 43 1 Introduction 1 1 Introduction Since the postulation of the concept of dark matter in the 1930s, more and more evidence for dark matter, such as astronomical observations, have been found and several theories have been developed in order to characterize it. However, none of these theories could be verified by an experimental detection so far, which makes it an interesting field of research. Most of these experimental setups look for a special class of theoretical particle, the Weakly Interacting Massive Particle (WIMP). One of these dark matter experiments following the path of detect WIMPs is the XENON Dark Matter Project, which is an international research project operating in the deep un- derground of the Laboratori Nazionali del Gran Sasso (LNGS) in Italy. The used detector, a dual phase xenon Time Projection Chamber (TPC), searches for WIMPs by looking for recoil signals. This detector setup, a combination of xenon as detection material and the dual phase concept, provides a high sensitive experiment for detecting WIMP-nucleon cross sections. The already operating development step, XENON100, which contains about 160 kg of liquid xenon, will be followed by the XENON1T detector, which further increases the sensitivity by 2 order of magnitudes. In order to investigate and test new systems for these experiments, as well as doing further studies of a similar detector setup in Muenster, a smaller scale TPC was built in 2011. This dual phase xenon Muenster TPC can be operated in the same way as the full scale experiments. For the calibration of the dual phase TPC experiments regarding its light yield (LY) and light collection efficiency (LCE), radioactive sources can be placed near the detector. The penetration of these sources depends on the inner volume of the detector, due to the self shielding effect of xenon, and on the used materials. The aim of this bachelor thesis is to investigate whether the available radioactive calibration sources can reach the entire detection volume or whether an alternative method, like an internal low energy calibration, can be used. In order to do this, a simulation package of the Muenster TPC was developed, and different calibration scenarios have been tested. After an introduction to the Muenster dual phase xenon TPC and GEANT4, the scintillation light propagation and the impact of different parameters on it, as well as LCE and LY map, will 2 1 Introduction be shown. Following this, the calibration possibilities of four different radioactive sources for the Muenster TPC will be presented. 2 The Muenster dual phase xenon time projection chamber 3 2 The Muenster dual phase xenon time projection chamber A typical time projection chamber (TPC) consists of a cylindric gas-filled detection volume, which is located in an electric or in some cases magnetic field, which can be applied to minimize the diffusion of electrons from ionization of the gas. The electric field is applied to drift the electrons to one side of the detector. This setup of a TPC allows to determine the primary position of the produced electrons. 2.1 Detector geometry and functionality The Muenster dual phase time projection chamber is a TPC which uses liquid xenon with a gaseous phase on top as active volume to detect scintillation and ionization signals (see [10]) created inside the liquid xenon by incoming particles. This dual phase xenon TPC consists of a cylindrical volume with a height of 170 mm and 80 mm diameter made of polytetrafluoroethylene (PTFE), better known as Teflon R . PTFE is chosen due to its high reflectivity for 178 nm photons, which are generated by interaction of different particles with liquid xenon [5]. The top and bottom of the detector volume is equipped with a total of 14 photomultiplier tubes (PMTs) for the detection of scintillation light. These photomultiplier tubes detect weak light signals due to the photoelectric effect, where electrons, called photoelectrons (pe), are emitted from a bialkali cathode due to the irradiation of photons, and amplified to a detectable signal. Incoming particles induce electron (photoexcitation and photoionisation) or nuclear recoils in the xenon and produce scintillation and ionization signals. The scintillation signal (S1) produced in the liquid volume is mainly reflected at the surface of the liquid xenon and detected by the bottom PMTs. Due to two externally applied electric fields, the electrons from the ionization drift along the 17 cm chamber until they reach the gas phase and are extracted into it by the second field. The first electric field from the bottom mesh, as shown in figure 2.1, set to negative potential (cathode), to the central mesh, set to ground (gate mesh), is called the drift field Edrift and has an electric field strength of 1 kV=cm. The second 4 2 The Muenster dual phase xenon time projection chamber Figure 2.1: Sketch of the working principle of the Muenster dual phase xenon time projection chamber. The origin of the S1 (scintillation) and S2 (scintillation due to ionized electrons) and the impact on the top PMT pattern are shown. The dimensions do not represent the real detector in every detail. electric field, applied between gate mesh and top mesh, set on positive potential (anode), is named extraction field Eextraction and has a field strength of 8:8 kV=cm. In order to protect the PMTs, two additional meshes are installed in front of each PMT pattern (see figure 2.1). The electrons in the gas phase are accelerated by the extraction field and produce a second scintillation signal, caused by interactions with xenon atoms in the gas phase (proportional scintillation), which is mainly collected by the top PMTs and is called S2 signal. The size of the S2 signal is directly proportional to the number of extracted electrons. As outlined above, a position reconstruction can be realized using the dual phase TPC. The depth of the interaction location (z-coordinate) can be determined by the drift time of the electrons from the ionization (see figure 2.1). These electron have a constant velocity of about 2 mm/µs [5]. As visualized in figure 2.1, the PMTs are arranged in an cylindrical 2-3-2 array. Due to this, the x- and y-coordinates can be calculated out of the hit pattern of the top PMTs. The quality of this full 3D position reconstruction is limited to the number of PMTs in the top array. While the resolution of the x- and y- coordinates is usually defined by the PMT shape and patterns, the z-position of the interaction, depending on drift velocity and drift time, can be obtained with a resolution of a few millimeters. 2 The Muenster dual phase xenon time projection chamber 5 Scintillation and ionization process According to [5], the scintillation process of xenon consists of different reactions. Put simply, ∗ the scintillation light is emitted by an excited xenon dimer state (Xe2) decaying to the ground state. This excitation state can be created in two different simultaneous reactions. The first reaction starts with an excited xenon atom, produced after scattering process with the incoming particle, as the initial condition. ∗ ∗ Xe + Xe + Xe ! Xe2 + Xe ∗ Xe2 ! 2Xe + hν (2.1) The second reaction is a collection of several reactions following an initial ionization: + + Xe + Xe ! Xe2 + − ∗∗ Xe2 + e ! Xe + Xe Xe∗∗ ! Xe∗ + heat ∗ ∗ Xe + Xe + Xe ! Xe2 + Xe ∗ Xe2 ! 2Xe + hν (2.2) As seen in these scintillation reactions, it can be outlined that xenon is transparent for its own scintillation photons since the produced photons are emitted by an excited dimer state, so that the scintillation light is not absorbed by other xenon atoms. The average required energy to produce one scintillation photon in liquid xenon is W = (21:61 ± 0:21) eV [11]. By applying the electric drift field, the second line in the reactions in equation (2.2) is suppressed and a part of the produced electrons is separated from the ions, thus missing for the recombination process. Due to the amplification of the ionization signal by the electric field the S2 signal is always much larger than the S1. With the ratio of S2=S1, which is always smaller for nuclear recoils than for electronic recoils, it is possible to perform a background discrimination of the electronic and nuclear recoil [5]. 2.2 Light collection efficiency and light yield As explained previously, the working principle of the Muenster dual phase xenon time pro- jection chamber is based on detecting scintillation light from interactions of particles with liquid xenon and accelerated electrons in the gaseous phase. According to this principle, the detection of light is one of the most important part of the detector setup.
Details
-
File Typepdf
-
Upload Time-
-
Content LanguagesEnglish
-
Upload UserAnonymous/Not logged-in
-
File Pages43 Page
-
File Size-