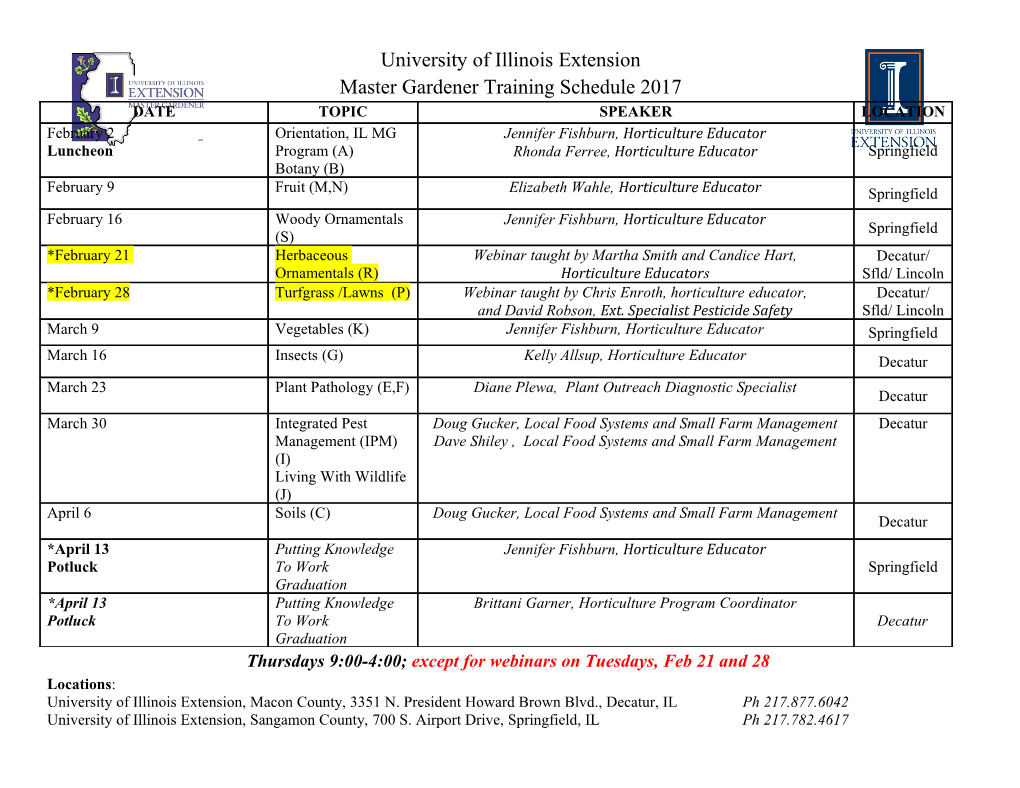
Development of stapled peptides targeting the Ral GTPases Catherine Hurd Department of Biochemistry University of Cambridge September 2020 This dissertation is submitted for the degree of Doctor of Philosophy Declaration This dissertation is the result of my own work and includes nothing which is the outcome of work done in collaboration except as specified in the Acknowledgements. It is not substantially the same as any that I have submitted, or, is being concurrently submitted for a degree or diploma or other qualification at the University of Cambridge or any other University or similar institution. I further state that no part of my dissertation has already been submitted, or is being concurrently submitted for any such degree, diploma or other qualification at the University of Cambridge or any other University or similar institution. It does not exceed the prescribed word limit of 60,000 words for the Degree Committee of the Faculty of Biology. i Acknowledgements Firstly, I would like to thank my supervisors, Darerca Owen and Helen Mott, for their generous support and guidance over the past four years. They have given a lot of their time to help me to develop as a researcher, and for that I am very grateful. I would like to thank AstraZeneca for funding this work and for allowing me to spend time on their site on Granta Park, which was a very valuable opportunity to see the inner workings of a pharmaceutical company. With thanks to Sarah Ross and Nicky Whalley, who have contributed to many useful discussions about the project and organized the provision of cell lines. Jefferson Revell was very generous with his time, providing training and advice for peptide synthesis at the AstraZeneca labs and during many useful and enthusiastic discussions about the project, for which I am very grateful. I would also like to thank the rest of the peptide lab who have always been so welcoming and helpful during my visits to Granta Park. The expertise of Paul Brear has been invaluable for all the work relating to the X-ray crystal structures, providing advice for crystallization conditions, carrying out the data acquisition and for solving the structures. In addition, Paul Brear and Katherine Stott have been very helpful in providing training and advice in the Biophysics facility. Many thanks also to Marc de la Roche and Jonathan Gadsby for assistance with confocal microscopy experiments. My time is Cambridge would not have been the same without all of the amazing members of the Mott/Owen group, who have made the lab a truly enjoyable place to work. Their friendship and support have been very important to me, and I thank them for the many hilarious coffee chats and pub trips over the past four years. I would also like to thank William Golding, who has been a rock to me for the past seven years and who is always able to find the positive in any situation. Finally, I owe so much to my Mum and Stepdad, Ruth and Simon Tobi, for their continued love and support. Writing this thesis during the national lockdown would have been so much more difficult without their wonderful company, and I will always treasure that time spent together. ii Abstract The ras genes are the most commonly mutated oncogenes in human cancers, with mutations occurring in approximately 20% of human tumours. However, more than 30 years of attempts to target Ras proteins therapeutically have yielded no effective therapies in the clinic, leading the proteins to be widely deemed ‘undruggable’. In recent years, there has been substantial evidence implicating the Ral GTPases, RalA and RalB, which are activated downstream of Ras, as critical drivers of cell growth and metastasis in numerous Ras-driven cancers. Therefore, targeting this pathway may provide an effective method for inhibition of oncogenic Ras signalling. Prior work identified stapled peptides based on the Ral effector, RLIP76, that can bind to the Ral GTPases and disrupt downstream signalling. To improve the affinity of these peptide sequences, an affinity maturation was performed on the Ral-binding domain of RLIP76 from which potential sequence changes were identified. The work described in this thesis aimed to identify sequences from this selection with improved affinity for Ral proteins to guide the design of second-generation stapled peptides targeting the Ral GTPases. In vitro validation of the selection sequences enabled the identification of several sequence substitutions that together improved binding to Ral proteins by more than 20-fold. The effects of individual residue substitutions on the affinity for Ral proteins were determined using biophysical assays and two 1.5 Å co-crystal structures of the tightest-binding mutants in complex with RalB revealed the key interactions formed. The sequences were successfully translated into stapled peptides based on RLIP76, resulting in peptides with improved affinity compared to the wild-type parent sequence. The peptides have been shown to be selective for the active form of Ral, with undetectable binding to a panel of related small GTPases in in vitro assays. The binding site of the lead peptide on RalB has been determined by NMR and was found to overlap with multiple Ral-effector interactions. The peptides were able compete with multiple Ral-effector interactions in vitro and in cellular lysates. This work demonstrates how manipulation of a native binding partner can assist in the rational design of stapled peptide inhibitors targeting a protein-protein interaction. iii Abbreviations ADP Adenosine diphosphate AEEP 9-Amino-4,7-dioxanonanoic acid Arf ADP ribosylation factor BSA Bovine serum albumin CD Circular dichroism Cdc42 Cell division control protein 42 homolog CPP Cell-penetrating peptide DCE 1,2-Dichloroethane DCM Dichloromethane DHFR Dihydrofolate reductase DIC N,N′-Diisopropylcarbodiimide DIPEA N,N-Diisopropylethylamine DMF Dimethylformamide ECL Enhanced chemiluminescence EDT 1,2-Ethanedithiol EDTA Ethylenediaminetetraacetic acid FAM 5-Carboxyfluorescein FKBP FK502-binding protein 12 FP Fluorescence polarization Fpa L-4-fluorophenylalanine FTase Farnesyltransferase FTI Farnesyltransferase inhibitor GAP GTPase activating protein GDI Guanine nucleotide dissociation inhibitor GDP Guanosine diphosphate Gdn-HCl Guanidine hydrochloride GEF Guanine nucleotide exchange factor GFP Green fluorescent protein GGTase Geranylgeranyltransferase GGTI Geranylgeranyltransferase inhibitor GMPPCP β,γ-Methyleneguanosine 5′-triphosphate GMPPNP Guanosine 5′-[β,γ-imido]triphosphate GST Glutathione S-transferase GTP Guanosine triphosphate 1-[Bis(dimethylamino)methylene]-1H-1,2,3-triazolo[4,5-b]pyridinium 3-oxide HATU hexafluorophosphate O-(1H-6-Chlorobenzotriazole-1-yl)-1,1,3,3-tetramethyluronium HCTU hexafluorophosphate iv HOBt Hydroxybenzotriazole HPLC High performance liquid chromatography HRV-3C Human Rhinovirus 3C IPTG Isopropyl β-d-1-thiogalactopyranoside ITC Isothermal titration calorimetry LC-MS Liquid chromatography-mass spectrometry MALDI-ToF MS Matrix-assisted laser desorption/ionization time of flight mass spectrometry MBP Maltose-binding protein MQ Milli Q (water) Nal D--naphthylalanine NMP N-Methyl-2-pyrrolidone NMR Nuclear magnetic resonance PBS Phosphate buffered saline PCR Polymerase chain reaction PDB Protein data bank PEG Polyethylene glycol PEI Polyethylenimine PMSF Phenylmethylsulfonyl fluoride POI Protein of interest PPI Protein-protein interaction PROTAC Proteolysis-targeting chimera Rab Ras-like in brain Ral Ras-like Ran Ras-like nuclear Ras Rat sarcoma RBD Ral binding domain Rho Ras homology RLIP76 Ral interacting protein 76 kDa RMSD Root-mean-square deviation SAW Sterile analytical water SDS Sodium dodecyl sulfate SDS-PAGE Sodium dodecyl sulfate–polyacrylamide gel electrophoresis SPA Scintillation proximity assay TEMED Tetramethylethylenediamine TFA Trifluoroacetic acid TIPS Triisopropylsilane v Contents 1 Introduction .............................................................................................................. 1 1.1 Ras superfamily of small GTPases..................................................................... 1 1.2 Common features of small GTPases ................................................................. 1 1.2.1 Regulation of small GTPases ..................................................................... 1 1.2.2 The G domain ........................................................................................... 3 1.2.3 The GTPase switch ................................................................................... 5 1.2.4 Membrane-targeting post-translational modifications ............................. 6 1.3 Differences in the structures of small GTPases ................................................. 7 1.4 Effector proteins .............................................................................................. 8 1.5 Ras proteins ................................................................................................... 10 1.5.1 Ras activation ......................................................................................... 11 1.5.2 Effector pathways .................................................................................. 12 1.5.3 Role of Ras proteins in cancer ................................................................ 14 1.6 Attempts to target Ras therapeutically .......................................................... 15 1.6.1 Disruption of membrane localization ..................................................... 16 1.6.2 Peptide inhibitors of Ras .......................................................................
Details
-
File Typepdf
-
Upload Time-
-
Content LanguagesEnglish
-
Upload UserAnonymous/Not logged-in
-
File Pages234 Page
-
File Size-