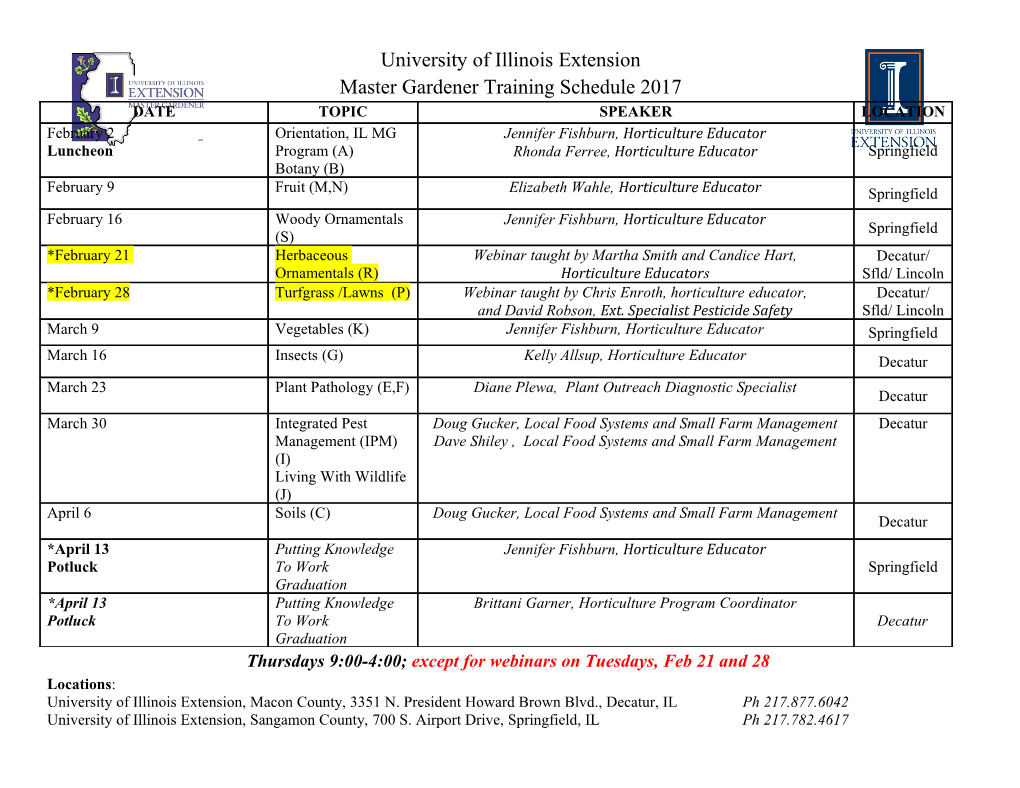
Seismic Evaluation of Differential Tectonic Subsidence, Compaction, and Loading in an Interior Basin1 Thomas H. Wilson2 ABSTRACT graphic intervals was nearly complete prior to deposition of later sequences. Local isostatic com- Quantitative analysis of depth-converted reflection pensation of differential loads across faults or fault times defines long-term differential motion across blocks requires movement along near-vertical crust- individual structures in a central Appalachian interior penetrating faults and abrupt thinning by differen- basin known as the Rome trough. Differential motion tial amounts across the base of the crust. This possi- decreases exponentially with time. Rotation about a bility seems unlikely within the context of current hinge defining the trough’s west margin reached models of intracratonic extension. Analysis indi- approximately 37% of total displacement in about cates that differential thickening of strata across 63–78 million years (m.y.). Displacement across the trough structures portrayed in reactivation history trough’s faulted east margin occurred more rapidly diagrams defines long-term tectonic movement and reached 37% of the total in 13–51 m.y. A major rather than a mixture of tectonic, compaction, and fault in the interior of the trough developed rapidly load-related motion. The analysis also suggests that with 37% of total displacement reached in from 16 to estimates of the time at which a given horizon 23 m.y. Longer term rotation across the west margin entered the oil or gas window and estimates of the may be due to its participation in the overall subsi- total depth reached by a horizon during subsidence dence of the craton during the Paleozoic. The time may also be in error if simple depth-dependent spanned by the formation of the East-Margin and compaction corrections are used. Interior faults was restricted to the Cambrian in the northern part of the area, but to the south, move- ment along the East-Margin fault continued through INTRODUCTION the Middle Ordovician. The general effects of differential compaction Modeling the tectonic development of sedimen- and loading for a single lithology model are com- tary basins has become an integral part of explo- puted from the standard compaction and Airy ration activities in the past two decades. Information isostasy equations. Depth-dependent compaction about the timing of deformation relative to the tim- requires that thicker strata over a hanging wall or ing of hydrocarbon migration is critical to deter- subsiding fault block undergo greater compaction mine if hydrocarbons will be trapped within a than occurs in thinner strata over the footwall or given structure. Deformation history of individual structurally high areas. Seismic interpretation of extensional structures within a basin is the net trough structures does not reveal the presence of result of tectonic and thermomechanical processes compaction faults or of long-term differential com- responsible for basin formation, combined with paction. The observations suggest that in this inte- lithosphere loading, sediment compaction, and rior basin differential compaction of major strati- variations of these factors throughout the basin (Ungerer et al., 1984). Models of basin development have become ©Copyright, 2000. The American Association of Petroleum Geologists. All rights reserved. increasingly complex. Sleep (1971) noted that an 1Manuscript received June 16, 1998; revised manuscript received June exponential thermal-cooling model accurately por- 22, 1999; final acceptance September 3, 1999. trays subsidence histories of the Atlantic continen- 2Department of Geology and Geography, West Virginia University, Morgantown, West Virginia 26506-6300; e-mail: [email protected] tal margin and several basins within the interior of This study was funded in part through U.S. Department of Energy the North American craton. The model derived by contract DE-AC22-90BC14657. Comments on earlier portions of this work by Robert Milici, Robert Shumaker, Byron Kulander, Lee Avary, Kevin Biddle, Sleep (1969) was initially used to explain mid-ocean and Jory Pacht were greatly appreciated. The paper also benefited from ridge topography as the result of thermal cooling of discussions with Robert Shumaker, Richard Beardsley, Joe Lemon, and isostatically compensated hot materials intruded Jovita Dominic. Appreciation is extended to Debbie Benson for her assistance in figure preparation. GTS Corporation provided some of the along the ridge axis. McKenzie (1978) noted that seismic data used in this study. simple thermal models require significant surface 376 AAPG Bulletin, V. 84, No. 3 (March 2000), P. 376–398. Wilson 377 Figure 1—Structure map of the central Appalachian foreland basin of North America. The locations of seismic lines analyzed in this study are shown (modified from Shumaker and Wilson, 1996). erosion of thermally uplifted regions to account for differential loading influence differential motion the elevated Moho observed beneath them. Using observed across faults and rotating fault blocks. the North Sea as an example, McKenzie (1978) noted that significant erosion has not been observed and suggests that stretching may have initially MAJOR BASEMENT STRUCTURE BENEATH thinned the crust. Sclater and Christie (1980) pre- THE STUDY AREA sented a detailed analysis in support of initial conti- nental stretching followed by thermal subsidence. Extension during the Early Cambrian deformed Long-term activity across fault zones and differ- the Precambrian foreland basement in the central entially subsiding fault blocks or margins is ob- Appalachian study area. The extensional basement served in seismic data. Yet, in the absence of complex formed by this event (Figure 2) is known detailed well-bore data required to specify porosity, as the Rome trough (McGuire and Howell, 1963) or density, lithology, and water depth across such the Eastern Interior aulacogen (Harris, 1978). The structures, apparent and continued displacement Rome trough is part of a larger system of grabens resulting from compaction and loading (respective- (Shumaker, 1986a, b; Thomas, 1991, 1993) that formed ly) cannot be estimated accurately and separated during the opening of the Early Paleozoic Iapetus from tectonic subsidence. In this paper, differential ocean. Several wells drilled in the area along with lim- motion across structures of the central Appalachian ited seismic data confirm the presence of the trough Rome trough (Figures 1, 2) is measured from seis- and a thick sequence of synrift rocks (e.g., Shumaker, mic profiles. Thickness variations computed from 1986a, b; Allen, 1988; Read, 1989; Ryder et al., 1992; depth-converted reflection traveltimes are used to Ryder, 1992; Shumaker and Wilson, 1996). describe relative motion across faults within the Interpretations of four seismic lines (Figure 1) complex as a function of geologic time. Compaction define major basement structure beneath the study and isostasy equations are solved to predict the area (Figure 2). An extensive normal fault (Figure 2) potential influence of compaction and loading for known as the East-Margin fault (Shumaker, 1993) generalized single-lithology models. Observations defines the east margin of the trough along its are compared to the generalized predictions. This length. With local exception, subsidence across the comparison is used to evaluate the degree to which western margin of the trough occurs through rota- sediment compaction and isostatic responses to tion about a hinge zone (Figure 2) known as the 378 Subsidence, Compaction, and Loading Figure 2—Contour map of acoustic basement across the Rome trough derived from lines 1 through 4. Ohio-West Virginia hinge zone (Ryder et al., 1992). that the trough, at regional scale, is a simple asym- In the southern part of the area along line 1 (Figures metrical graben. The western limb gradually subsid- 2, 3) the trough forms a simple asymmetrical graben; ed relative to the Ohio-West Virginia hinge zone that however, to the north the trough widens and is sub- lies off the line to the northwest (Figure 2). The East- divided by one or more interior faults. Minor inter- Margin fault forms the eastern boundary of the nal faults are ubiquitous within the trough interior trough, although minor structures are observed far- and are not represented at this scale. ther east in the footwall on line 1 (Figure 3). Minor Sonic logs from deep wells in the area (see structural irregularity is observed within the trough Shumaker and Wilson, 1996) were used for velocity (Figure 3). A low-relief structural high is present control. A constant velocity function derived from across the trough in the Silurian Rose Hill through the sonic logs was used to convert reflection travel- Devonian Huron reflections (Figures 3, 5). Depth- time to depth. There are a limited number of sonic converted seismic traveltimes reveal a structural rise logs in the region, but comparison between them of of approximately 100 m in the Devonian Onondaga the average velocities for major stratigraphic inter- reflector west of the East-Margin fault into the inte- vals does not reveal significant velocity variation. rior of the trough. Structural relief observed on con- Line 1 (Figure 3) is a reprocessed single-fold seismic tour maps of well log data presented by Gao and line, whereas lines 2, 3, and 4 are multichannel (opti- Shumaker (1996) of the Onondaga Limestone in mal 24-fold) Vibroseis™ data. The quality of the data this area also reveal approximately
Details
-
File Typepdf
-
Upload Time-
-
Content LanguagesEnglish
-
Upload UserAnonymous/Not logged-in
-
File Pages23 Page
-
File Size-