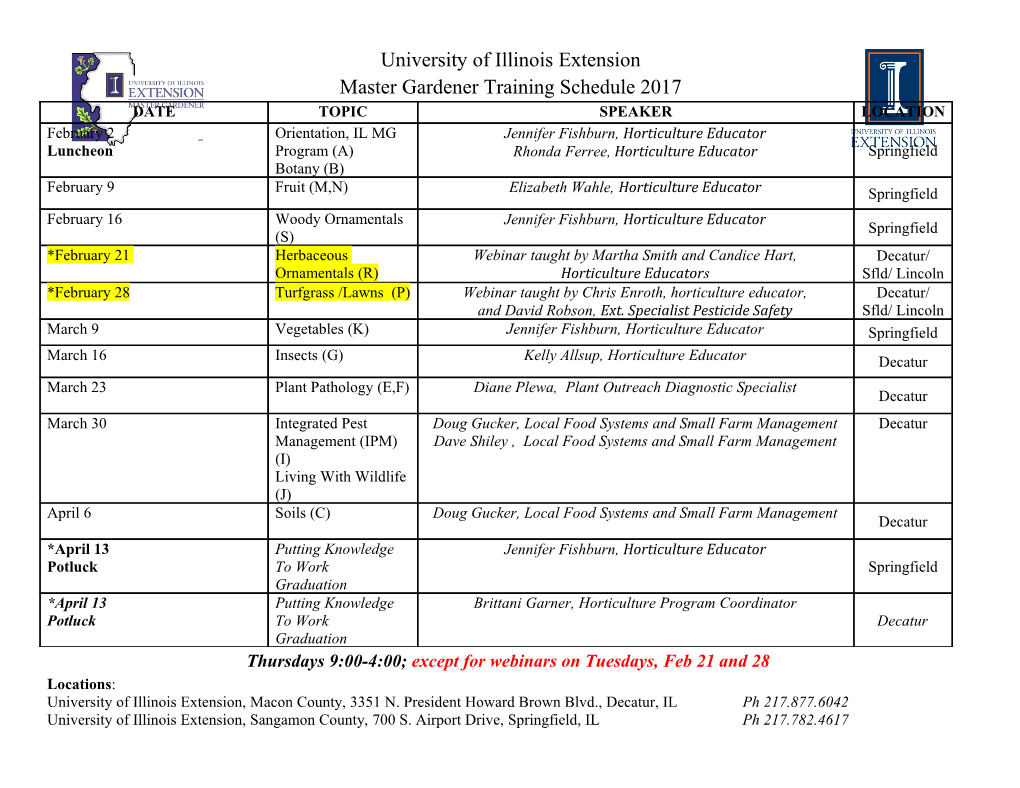
This is an open access article published under a Creative Commons Attribution (CC-BY) License, which permits unrestricted use, distribution and reproduction in any medium, provided the author and source are cited. Letter Cite This: J. Phys. Chem. Lett. 2019, 10, 2829−2835 pubs.acs.org/JPCL Analysis of the Voltage Losses in CZTSSe Solar Cells of Varying Sn Content Mohammed Azzouzi,†,# Antonio Cabas-Vidani,‡,# Stefan G. Haass,‡ Jason A. Röhr,† Yaroslav E. Romanyuk,‡ Ayodhya N. Tiwari,‡ and Jenny Nelson*,† † Department of Physics and Centre for Plastic Electronics, Imperial College London, London SW7 2AZ, United Kingdom ‡ Laboratory for Thin Films and Photovoltaics, Empa-Swiss Federal Laboratories for Materials Science and Technology, Ueberlandstrasse 129, 8600 Duebendorf, Switzerland *S Supporting Information ABSTRACT: The performance of kesterite (Cu2ZnSn- (S,Se)4, CZTSSe) solar cells is hindered by low open circuit fi voltage (Voc). The commonly used metric for Voc-de cit, namely, the difference between the absorber band gap and fi qVoc,isnotwell-dened for compositionally complex absorbers like kesterite where the bandgap is hard to determine. Here, nonradiative voltage losses are analyzed by measuring the radiative limit of Voc, using external quantum efficiency (EQE) and electroluminescence (EL) spectra, without relying on precise knowledge of the bandgap. The method is applied to a series of Cu2ZnSn(S,Se)4 devices with Sn content variation from 27.6 to 32.9 at. % and a corresponding Voc range from 423 to 465 mV. Surprisingly, ffi the lowest nonradiative loss, and hence the highest external luminescence e ciency (QELED), were obtained for the device with ff the lowest Voc. The trend is assigned to better interface quality between absorber and CdS bu er layer at lower Sn content. esterite solar cells are a promising earth-abundant poor and Zn-rich. This reduces the formation of detrimental fi K alternative to existing thin lm photovoltaic technolo- defects like interstitials (Cui and Zni) and [2CuZn +SnZn] gies.1 Even though their power conversion efficiencies (PCE) antisite clusters that adversely affect the solar cell performance, have increased significantly during the past decade from 4% in while increasing the concentration of copper vacancies (VCu), 2004 to 12.6% in 2014,2 they still lie far below those of which is a beneficial shallow acceptor.15,16 Downloaded via LIB4RI on November 26, 2019 at 07:21:09 (UTC). competing technologies like Cu(In,Ga)Se2 and CdTe that have It has been shown that CZTSSe absorbers and related 3 fi − surpassed 22%. One of the major issues is the signi cant Voc sulfur selenium alloys crystallize in the kesterite-type structure fi fi de cit in these devices de ned relative to the bandgap Eg of the where the band gap varies from 1.0 eV for pure selenide − absorber or relative to the Voc in the Shockley Queisser limit (CZTSe) to 1.5 eV for pure sulfurized (CZTS), opening the 4 − for the same band gap (Voc,SQ(Eg)). Kesterite devices show a possibility to tailor the band gap of kesterite through the S Se See https://pubs.acs.org/sharingguidelines for options on how to legitimately share published articles. fi − de cit (Eg/q Voc) larger than 0.55 V whereas competing content. Additionally, the structural instability due to the low technologies have reduced these losses to less than 0.4 V.5 In enthalpy cost of swapping Cu and Zn atoms in kesterites has fi ff 17 the literature, the lowest values of Voc de cit in devices have also been shown to a ect the band gap. In fact, the ordered been obtained by means of interfacial and compositional CZTSe (Cu2ZnSnSe4) has a band gap 100 meV higher than 6,7 18 ́ optimization with the highest achieved Voc relative to the the disordered one. Marquez et al. observed that Cu-poor band gap of a kesterite solar cell being still 0.4 V lower than CZTSe had a higher band gap and Voc, linked to an increased 8 V . ordering of the Cu/Zn sublattice, while the Voc,def remained oc,SQ 19 Several mechanisms have been investigated as the origin for constant. Similarly, through annealing procedures with − the large voltage losses in CZTSSe solar cells.9 11 A primary increasing temperatures and subsequent rapid cool-down, a − mechanism is thought to be related to the high defect density reversible order disorder transition was shown to occur at a 12,13 ° and associated band tailing of CZTSSe materials, which critical temperature of about 200 C, leading to a band gap shift equivalent to the one observed with the change in Cu are attributed to the multielement composition nature of the 20 quaternary CZTSSe phase. The similar ionic radii, and content. comparable valences of elements like copper and zinc, lead to a narrow chemical stability region and multiple defects with Received: February 21, 2019 low activation energies.14 The best performing devices have an Accepted: May 9, 2019 off-stoichiometric absorber composition, more specifically Cu- Published: May 9, 2019 © 2019 American Chemical Society 2829 DOI: 10.1021/acs.jpclett.9b00506 J. Phys. Chem. Lett. 2019, 10, 2829−2835 The Journal of Physical Chemistry Letters Letter The strong dependence of the band gap of kesterite kT JVph,SQ ()oc absorbers on their composition and processing condition, V =+B ln 1 oc,SQ q J coupled with the complexity of quantifying the band gap 0,SQ (2) 21 energy of a disordered system such as CZTSSe, raises the i y question of whether a V loss analysis solely related to the where Jph,SQ is the photocurrentj densityz in the radiative limit oc and J is the SQ darkj saturation currentz density. In practice, band gap energy gives relevant information concerning the 0,SQ j z origin of the losses and their dependence on material Jph,SQ(Voc) can bek replaced by the{ measured short-circuit current density (J ) at the corresponding light intensity. As properties. From the reciprocity principle and the measured sc discussed in ref 23, this assumption introduces a small error in external quantum efficiency, Rau et al.22 introduced a radiative the calculation of the open circuit voltages of some millivolts limit for the open circuit voltage that incorporates the actual that can be neglected. The J is obtained directly from the absorption tail of the materials, as expressed via the external 0,SQ ffi band gap Eg following the detailed balance of absorption and quantum e ciency (EQE) spectrum. On the basis of this work, emission in solar cell as expressed by Shockley and Queisser:4 Yao et al. introduced another way to quantify the losses in solar 23 ff ∞ cells, where they di erentiated the losses due to an extended Jq= ϕ ()d EE Δ 0,SQ ∫ BB absorption onset ( Voc,abs) from the losses due to nonradiative Eg (3) recombination (ΔV ), that are dependent on the ratio of oc,nrad where a step function for the external quantum efficiency at the radiative to nonradiative recombination rates. This approach ϕ band gap is assumed. BB is the spectral blackbody emission was used on organic solar cells to distinguish nonradiative fl losses from the total losses to indicate how changes in material ux density in the forward direction integrated from the 24,25 surface of the blackbody at the cell temperature Tc or processing could bring Voc closer to the radiative limit. In this work we focus on a series of CZTSSe solar cells E2 1 where compositional variation, namely, the Sn content, was ϕπBB(,)ET =× 2 32 ff hc E shown to a ect Voc, as well as the band gap energy of the exp− 1 ()()kTBc (4) absorber in a similar manner to the ordering effect of the Cu/ fi −2 −1 −1 ’ Zn sublattice. We rst show how a method relying solely on Eg which is expressed in units of cm s eV . Here h is Planck s fi to quantify the Voc de cit depends strongly on the way Eg is constant and c is the speed of light in vacuum (we consider the calculated. We then use the method described above refractive index of the external medium surrounding the cell to 23 26 developed by Yao et al. to calculate the radiative limit of be ns = 1). Using a similar approach, but considering the ffi the voltage (Voc,rad). This approach has never been applied to actual quantum e ciency of the cell (EQE(E)), we can express CZTSSe absorbers, but it proves to be very relevant, since the the radiative dark saturation current J0,rad as the integral of the bandgap cannot be reliably determined by traditional methods, EL emission from the cell 21 fi due to material complexity. Surprisingly, we nd that this Voc Jq==ϕϕ()d EEqEE EQE() ()dE increase with Sn content was accompanied by an increase 0,rad ∫∫EL BB (5) Δ ff rather than a decrease of Voc,nrad, indicating a negative e ect ϕ fl where EL is the spectral photon ux density emitted by the of the Sn content on the nonradiative relative to radiative ’ ϕ cell. We used Rau s reciprocity principle to write EL as recombination rate. By measuring both temperature dependent ϕ 22 EQE BB for the cell at equilibrium. Away from equilibrium, current voltage characteristic (JV-T) and temperature depend- δϕ ent capacitance-frequency (Cf−T), we discard the hypothesis the excess electroluminescence emission ( em(E)) is related to the internal voltage V experienced by the cell that this improvement is due to a change in the defect int distribution in the absorber structure and show that it can be qVint related to the interfacial quality between the absorber and the δϕϕem(EEET )=− EQE( ) BB ( , ) exp 1 buffer layers.
Details
-
File Typepdf
-
Upload Time-
-
Content LanguagesEnglish
-
Upload UserAnonymous/Not logged-in
-
File Pages7 Page
-
File Size-