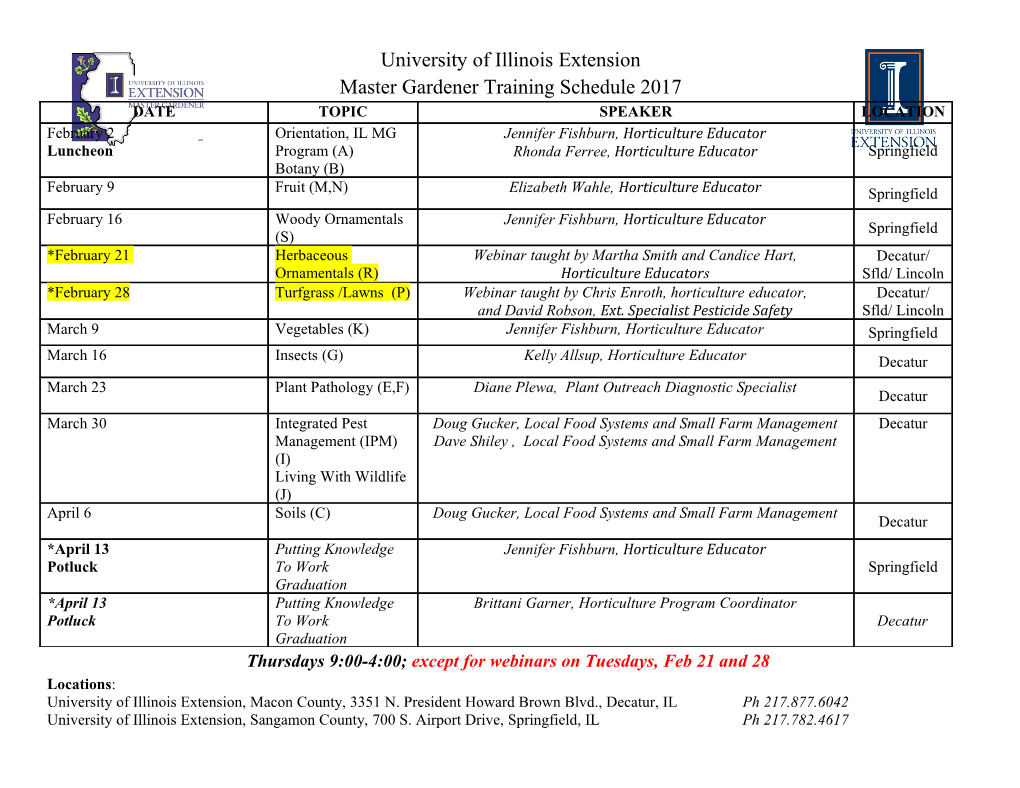
Overview of Multi-Frequency EPR Most EPR (Electron Paramagnetic Resonance) experiments are performed at X-band (9-10 GHz). Many factors contribute to the choice of this frequency. One factor is the availability of components: with the development of RADAR and the end of World War II, there was a glut of surplus X-band mil- itary microwave equipment available to the early EPR pioneers. In addition to historic factors, convenience plays an important role. The mag- netic field requirements for X-band are usually satisfied easily by electro- magnets. The 3 cm wavelength ensures convenient sample sizes and sample handling. A third consideration is sensitivity. As the Boltzmann factor and sample size increase, they increase the spectrometer’s sensitivity. Alas, the two factors are not independent. The Boltzmann factor increases with increasing frequency, but the improvement in sensitivity is tempered by the decrease in sample size with increasing frequency. If you have sufficient sample, albeit at a low con- centration, X-band often offers the best sensitivity. These factors lead to the almost universal acceptance that EPR is performed at X-band. Given all the advantages of X-band EPR, why would researchers wish to perform experiments at lower or higher frequencies? Performing EPR spectroscopy at multiple frequencies sheds additional light on the properties of the sample. If we were to see everything only in black and white, we would miss all the extra information that color vision affords us. In an analogous fashion, if we were to measure our samples at X-band only (black and white), we would miss the complete picture that other microwave frequencies (color vision) could offer us. The appearance of EPR spectra depends strongly on the interplay of magnetic field dependent and magnetic field independent interactions. By operating at several frequencies, we are able to resolve the contributions from the two types of interactions and thereby obtain unambiguous answers to the ques- tions posed by our sample. In this overview we shall consider the advantages of going higher or lower in frequencies compared to X-band. We shall explore the benefits from the com- plementary information offered by high and low frequencies. Finally, the methodology and technical aspects of multi-frequency EPR spectroscopy will be introduced. Overview of Multi-Frequency EPR Some Preliminary Definitions Some Preliminary Definitions 1 This overview will make use of certain terms that may not be common knowledge for many people using EPR spectroscopy. Here we shall define some of those terms. Multi-frequency EPR embodies the use of high and low microwave frequen- cies in studying paramagnetic samples. High frequency is defined as micro- wave frequencies above X-band (~10 GHz). Low frequency is defined as microwave frequencies below X-band. Frequencies are often identified by the waveguide size known as frequency bands. Table 1 lists the most commonly used bands. In EPR, the definitions sometimes differ from the electrical engi- neering definitions. Column 3 shows the frequencies used by Bruker Biospin Corporation for some of the frequency bands. Common Microwave Frequency Microwave Frequency for EPR Band Frequencies (GHz) (GHz) L 1-2 ~1 S 2-4 ~4 C 4-8 X 8.2-12.4 9.2-9.9 Ku 12.4-18 K 18-26.5 ~24 Q 26.5-40 ~34 V 40-75 W 75-110 ~94 D 110-170 Table 1 Frequencies for commonly used microwave bands. Frequencies lower than 1 GHz are often designated as VHF (the seemingly incongruous term Very High Frequency). Frequencies above 18 GHz are often labeled as millimeter waves because the wavelength is approaching 1 cm or less. The microwave frequencies commonly used in EPR were not chosen arbi- trarily. With the exception of K-band, the common EPR frequencies corre- spond to minima in the atmospheric absorption of microwaves. (See Figure 1.) This has the advantage that systems do not have to be purged to displace oxygen or water vapor in the waveguide and resonator. In addition, there are more components available at these frequencies because these are the frequencies that would be used for RADAR and communications pur- poses. 2 Why Use Multiple Frequencies? Average Atmospheric Absorption of Millimeter Waves 100 40 20 10 D-band HO HO 2 ) 2 m 4 K O / 2 B 2 d W-band ( n 1 o i t a Q-Band u O n 0.4 2 e t t A 0.2 0.1 X-Band 0.04 HO2 0.02 10 15 20 25 30 40 50 60 70 80 90100 150 200 250 300 400 Frequency (Ghz) Figure 1 Atmospheric absorption of microwaves at different frequen- cies. Why Use Multiple Frequencies? 2 In the introduction, the importance of magnetic field dependent and magnetic field independent interactions was briefly mentioned. Here, we shall expand on this idea. Often, a spin-hamiltonian of the form: H = eS g B0 + S D S + S A I [1] } } magnetic magnetic field field dependent independent describes the EPR spectrum well. The symbols have the following meanings: • e = Bohr magneton • S and I = electronic and nuclear spin operators • g = electronic g matrix • B0 = the externally applied magnetic field • D = the ZFS (Zero Field Splitting) matrix • A = the nuclear hyperfine matrix Because they are often weaker than electronic Zeeman and hyperfine interac- tions, we shall not consider the nuclear Zeeman and quadrupolar interactions. The first term in the spin-hamiltonian is the electronic Zeeman interaction and owing to the B0 variable, this is the magnetic field dependent term of the expression. The second two terms, the ZFS and nuclear hyperfine interac- tions, are the magnetic field independent terms. The ZFS term only contrib- utes for spin systems where S>1/2. Overview of Multi-Frequency EPR 3 The Effects of g-Values Each of the spin-hamiltonian parameters offers us specific information about our sample. The g-value can help identify a paramagnetic species as well as tell us about the electronic state and symmetry of the paramagnetic site. The ZFS terms inform us about the spin and valence state as well as symmetry of paramagnetic centers. The nuclear hyperfine interactions supply us with iden- tity, number, and distances of surrounding nuclei. Therefore, each of the spin-hamiltonian parameters gives important and different information. Ideally, we would like to obtain an EPR spectrum in which the spin-hamilto- nian parameters are evident from visual inspection of the spectrum’s features. Alas, several factors such as linewidths and anisotropy may mask the features we wish to interpret. In addition, second-order* effects can make interpreta- tion difficult. Higher magnetic fields make the electronic Zeeman term more dominant, thereby suppressing second-order effects and making the spectra more first-order. Simulations with least-squares analysis may yield the desired spin-hamiltonian parameters in such cases, however, the parameters obtained may not be unique values or an unambiguous interpretation of the spectrum. One solution to this problem is to perform EPR experiments at different fre- quencies. By using different frequencies, we establish different magnetic fields for resonance from our sample and thereby we emphasize or accentuate the effects of the magnetic field dependent or independent terms of our spin- hamiltonian on our EPR spectrum. By going to very low and very high fre- quencies, we can achieve limiting cases where magnetic field independent or dependent effects dominate, offering us the opportunity to unambiguously measure the parameters we need. Even if we cannot measure our sample in these limiting conditions, having data at several frequencies often supplies the needed constraints to successfully and unambiguously obtain the spin-hamiltonian parameters from simulation and simultaneous least-squares minimization of the multi-frequency EPR data. The Effects of g-Values 3 Our NMR colleagues have been exploiting increasingly higher fields and fre- quencies in order to attain increasingly better resolution. The higher frequen- cies accentuates the chemical shift to the point where the shift is greater than the linewidth. The corresponding spin-hamiltonian parameter for EPR is the isotropic g-value. In the absence of other interactions, the field for resonance is given by: h B = -------- [2] 0 g e * First order means that the electronic Zeeman interaction is much greater than the other interactions and they contribute simple corrections to the energy levels. Second order means that the electronic Zeeman interaction is only slightly greater than the other interactions and they contribute large and more complicated corrections to the energy levels. 4 The Effects of g-Values where h is Planck’s constant, is the microwave frequency, g is the isotropic g-value, and e is the Bohr magneton. If we were to have two paramagnetic species in our sample with a small g-value difference of g, the difference in fields for resonance, B, is approximately proportional to the microwave fre- quency: h g B = ------------------------------ . [3] egg+ g If B can be made greater than the linewidth by increasing the microwave frequency, we can unambiguously identify the two species in our sample. Figure 2 shows such an example. The sample consists of a mixture of two nitroxides (proxyl and TEMPOL) with slightly different g-values. At X-band, the broad small shoulder on the low field line hints that there may be two spe- cies. By repeating the experiment at higher frequency (W-band), the signals from the two species are sufficiently split that we can unambiguously say there are two species. In addition, the resolution is sufficient that we can even measure the relative concentrations of the two species. X-Band W-Band Figure 2 Using a higher microwave frequency to resolve nitroxides (proxyl and TEMPOL) with slightly different g-values.
Details
-
File Typepdf
-
Upload Time-
-
Content LanguagesEnglish
-
Upload UserAnonymous/Not logged-in
-
File Pages18 Page
-
File Size-