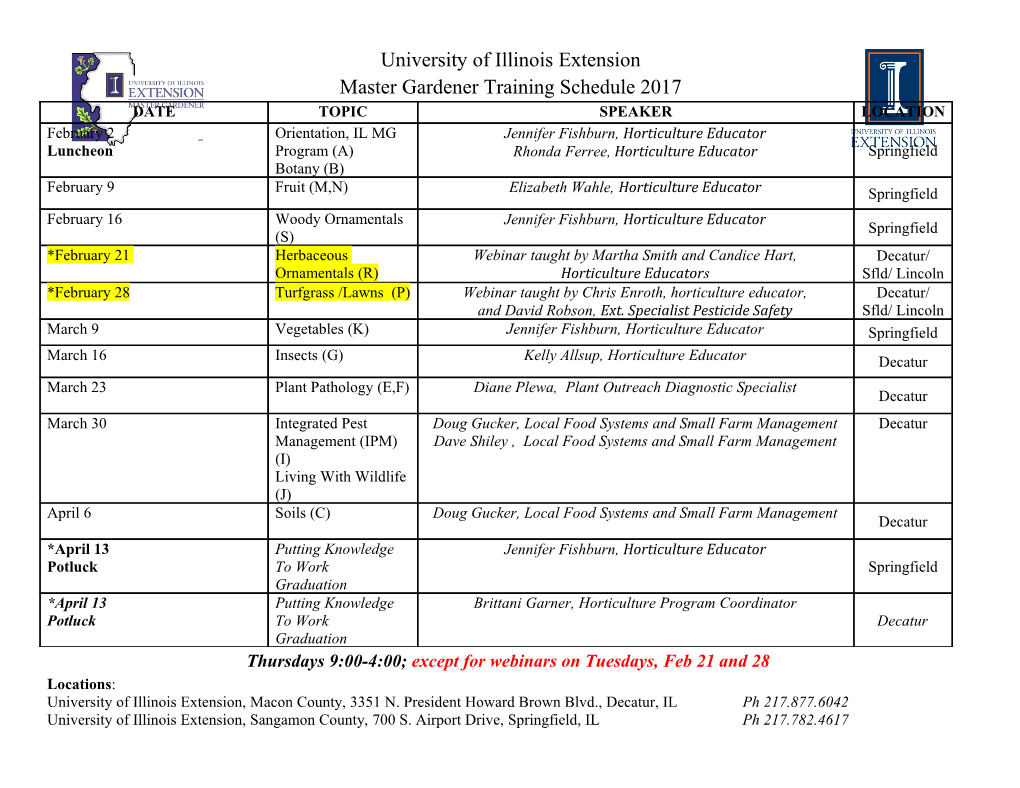
Resonant Wireless Power Transfer with Embedded Communication for More Versatile and Efficient Applications by Erik Johnson Submitted to the Department of Electrical Engineering and Computer Science in partial fulfillment of the requirements for the degree of Master of Engineering in Electrical Engineering and Computer Science at the MASSACHUSETTS INSTITUTE OF TECHNOLOGY June 2016 ○c Erik Johnson, MMXVI. All rights reserved. The author hereby grants to MIT permission to reproduce and to distribute publicly paper and electronic copies of this thesis document in whole or in part in any medium now known or hereafter created. Author................................................................ Department of Electrical Engineering and Computer Science May 20, 2016 Certified by. Eko Lisuwandi Senior Design Engineer, Linear Technology Thesis Supervisor Certified by. David Perreault Professor of Electrical Engineering Thesis Supervisor Accepted by........................................................... Dr. Christopher J. Terman Chairman, Masters of Engineering Thesis Committee 2 Resonant Wireless Power Transfer with Embedded Communication for More Versatile and Efficient Applications by Erik Johnson Submitted to the Department of Electrical Engineering and Computer Science on May 20, 2016, in partial fulfillment of the requirements for the degree of Master of Engineering in Electrical Engineering and Computer Science Abstract This thesis explores the feasibility of embedding communication within the power transfer channel of a near field wireless power transfer system in order to increase the versatility and efficiency of the system. The system was built around the LTC4125 auto-resonant wireless power transmitter IC. Both the transmitter and receiver were tuned to approximately 100kHz with a communication data bit rate of 1kHz. The thesis covers both resonant wireless power transfer theory, the details of the embedded communication and the results obtained. Thesis Supervisor: Eko Lisuwandi Title: Senior Design Engineer, Linear Technology Thesis Supervisor: David Perreault Title: Professor of Electrical Engineering 3 4 Acknowledgments I would like to first thank my 6A advisor Eko Lisuwandi for his willingness towork with me and be my advisor. I am thankful for his guidance and patience throughout the project. I enjoyed discussing different obstacles with him and I appreciate that he was always available to help me think about any problem. I would like to thank Sam Nork at Linear for making this great opportunity possible. I would like to thank Ron Swinnich for his help in my moments of need and his unfailing company in the lab. I would also like to thank the other engineers at Linear for creating a very welcoming and enjoyable work environment. I especially am thankful for Erjon, Maziar and Chris and all of the fun we had playing soccer together. I would like to thank Professor David Perreault for his willingness to my on-campus advisor and for being a great mentor during my senior year UROP. I would like to thank my friends at MIT for the countless memories, their encour- agement and friendship throughout my time at MIT. I would like to thank my parents and my family for their enduring love and support. The sacrifices they have made and the love they have shown me has made it all possible. Finally I am thankful to God for the uncountable blessings I am given each day. 5 6 Contents 1 Near Field Resonant Wireless Power Transfer 19 1.1 Overview of Near Field Wireless Power . 19 1.1.1 Near Field Wireless Power Transfer . 19 1.1.2 Resonant Inductive Power Transfer . 20 1.2 Use Applications . 20 1.3 Optimization Methods . 21 1.3.1 Optimization without Adaptive Control . 21 1.3.2 Optimization with Adaptive Control . 23 1.4 Mathematical Modeling . 28 1.4.1 Basic Terminology . 28 1.4.2 Linear Models of Resonant Power Transfer . 31 1.4.3 Voltage Transfer Functions and Peak Splitting . 36 1.4.4 Linear Model Efficiency Derivation . 48 1.5 LTC Wireless Charging Options . 54 1.5.1 LTC 4120 . 54 1.5.2 LTC 4125 . 56 2 Rectifier Modeling, Initial Data Measurements and Thesis Objec- tives 57 2.1 Rectifier Model . 57 2.1.1 Buck Converter . 60 2.1.2 Effective Load . 60 2.2 Initial Data Measurements . 61 7 2.2.1 Verifying the Linear Model with Data Measurements . 61 2.2.2 AC Load Efficiency . 62 2.2.3 Variable Rectified Voltage Measurements . 63 2.3 Identification of Thesis Objectives . 72 3 Communication 73 3.1 Communication overview . 73 3.2 Description of the Uplink Communication: Power Receiver to Power Transmitter . 74 3.2.1 Differential Manchester Encoding . 75 3.3 Description of the Downlink Communication: Power Transmitter to Power Receiver . 84 3.4 Signal Processing . 87 3.4.1 Analog Filtering . 87 3.4.2 Digital Filtering . 91 4 Feedback Control Theory and the Closed Loop System 93 4.1 Modeling a Continuous Time System as a Discrete Time System . 93 4.2 Modeling a Continuous Time Controller in Discrete Time . 96 4.2.1 Discrete Time Filters . 99 4.3 Open Loop System . 101 4.4 Smith Predictor Controller . 102 4.5 Closed Loop System . 106 4.5.1 Block Diagram and Calculations . 106 4.5.2 Measurements . 110 5 Battery Charging 113 5.1 Overview of Battery Charging . 113 5.2 Analog Controlled Battery Charging . 114 5.3 Digitally Controlled Battery Charging . 116 8 6 Communication and Max Efficiency Finding Algorithm 117 6.1 Efficiency Optimization Overview . 117 6.2 Power Transmitter Side Flow Chart . 118 6.3 Power Receiver Side Flow Chart . 120 6.4 Power Transmitter Efficiency Optimization Flow Chart . 124 7 Results and Future Considerations 127 7.1 Efficiency Comparisons . 127 7.2 Range Extension . 131 7.3 Future Considerations to Improve the System . 132 A Pictures of the System 135 B System Code 137 B.1 Power Transmitter Code . 137 B.2 Power Receiver Code . 202 9 10 List of Figures 1-1 Capacitor Modulation Circuit . 24 1-2 Voltage waveforms on the primary resonator of a load-impedance mod- ulated signal . 24 1-3 Standard Communication Diagram . 25 1-4 Frequency Response of a resonant LC circuit. The modulation side- bands are shown at the frequency of the carrier plus and minus half bandwidth . 26 1-5 A system with seperate inuctuve power and data links. 27 1-6 Linear Model with a Parallel Receiver Tank . 32 1-7 Feedback Block Diagram for the Linear Model . 33 1-8 Linear Model with a Series Receiver Tank . 35 1-9 Parallel Resonant Voltage TF vs. Frequency for R = 10 Ohms and Various Coupling Factors . 37 1-10 Parallel Resonant Voltage TF vs. Frequency for R = 50 Ohms and Various Coupling Factors . 38 1-11 Parallel Resonant Voltage TF vs. Frequency for R = 100 Ohms and Various Coupling Factors . 38 1-12 Unloaded Root Locus for a Parallel Resonant Receiver . 39 1-13 Root Locus for a Parallel Resonant Receiver with R = 100 . 40 1-14 Series Resonant Voltage TF vs. Frequency for R = 10 Ohms and Various Coupling Factors . 41 1-15 Series Resonant Voltage TF vs. Frequency for R = 50 Ohms and Various Coupling Factors . 41 11 1-16 Series Resonant Voltage TF vs. Frequency for R = 100 Ohms and Various Coupling Factors . 42 1-17 Root Locus for a Series Resonant Receiver with R = 10 . 43 1-18 Parallel Off-Resonant Voltage TF vs. Frequency for R = 10 Ohms and Various Coupling Factors . 44 1-19 Parallel Off-Resonant Voltage TF vs. Frequency for R = 50 Ohms and Various Coupling Factors . 44 1-20 Parallel Off-Resonant Voltage TF vs. Frequency for R = 100 Ohms and Various Coupling Factors . 45 1-21 Series Off-Resonant Voltage TF vs. Frequency for R = 10 Ohms and Various Coupling Factors . 46 1-22 Series Off-Resonant Voltage TF vs. Frequency for R = 50 Ohms and Various Coupling Factors . 46 1-23 Series Off-Resonant Voltage TF vs. Frequency for R = 10 Ohms and Various Coupling Factors . 47 1-24 Parallal Resonant Efficiency . 51 1-25 Series Resonant Efficiency . 52 1-26 Parallel vs Series Resonant Efficiency . 53 1-27 Effective Resistance of Battery Charging for Various Rectified Voltages 53 1-28 LTC4120 Diagram . 55 2-1 Resonant DC-DC Converter . 58 2-2 Power Receiver Side with a Half Bridge Rectifier . 58 2-3 Wireless Power Circuit with a Rectified and Bucked Load . 61 2-4 Linear Model Verification . 62 2-5 Predicted vs Measured Efficiencies for an AC Load . 63 2-6 Efficiency vs Output Power for Various Rectified Voltages with k=0.41 64 2-7 Efficiency vs Output Power for Various Rectified Voltages with k=0.13 65 2-8 Output Power vs Rect V for Various Input Duty Cycles and K = 0.13 66 2-9 Output Power vs Rect V for Various Input Duty Cycles and K = 0.188 66 12 2-10 Output Power vs Rect V for Various Input Duty Cycles and K = 0.41 67 2-11 Output Power vs Duty Cycle for Various Rect V and k = 0.188 . 68 2-12 Output Power vs Duty Cycle for Various Rect V and k = 0.41 . 69 2-13 Efficiency vs Rect V for Various Input Duty Cycles and K =0.41. 70 2-14 Efficiency vs Rect V for Various Input Duty Cycles and K =0.28. 70 2-15 Efficiency vs Rect V for Various Input Duty Cycles and K = 0.188. 71 2-16 Efficiency vs Rect V for Various Input Duty Cycles and K =0.13. 71 3-1 Basic Communication Diagram . 74 3-2 Capacitor Modulation Circuit . 75 3-3 Differential Manchester Encoding . 75 3-4 Screenshot of Communication . 76 3-5 Screenshot of Decoding the Uplink Communication . 78 3-6 Decoding the Uplink Communication .
Details
-
File Typepdf
-
Upload Time-
-
Content LanguagesEnglish
-
Upload UserAnonymous/Not logged-in
-
File Pages240 Page
-
File Size-