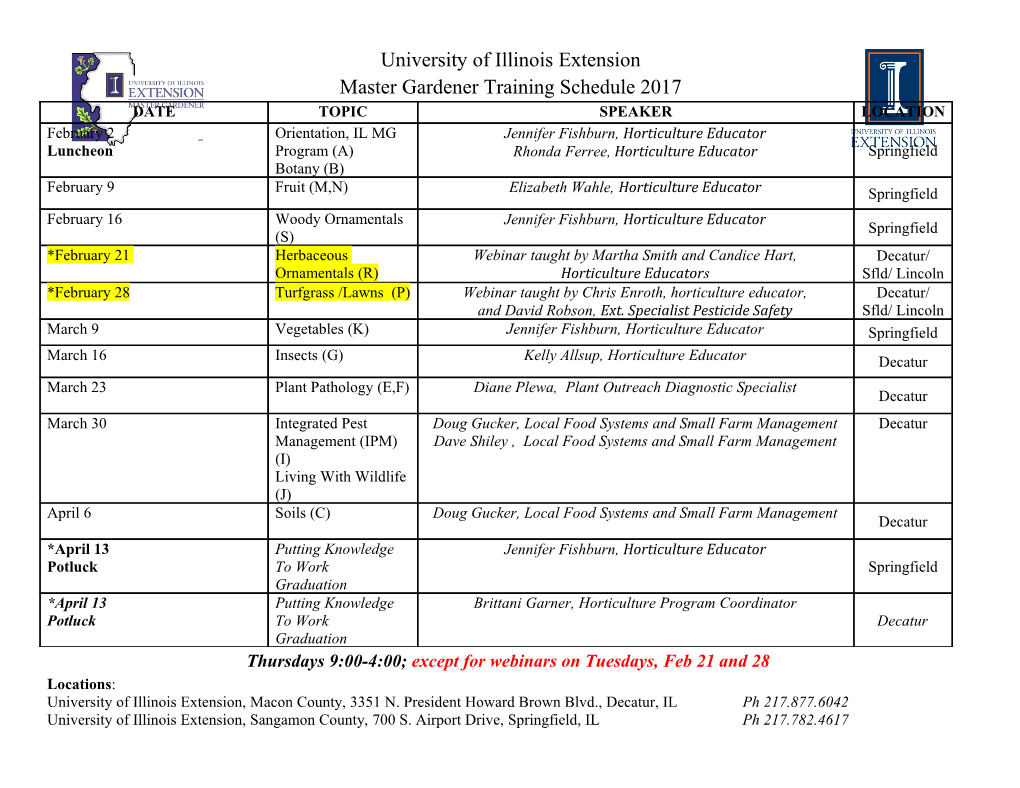
Protein shape modulates crowding effects Alex J. Gusemana, Gerardo M. Perez Goncalvesa, Shannon L. Speera, Gregory B. Youngb, and Gary J. Pielaka,b,c,d,1 aDepartment of Chemistry, University of North Carolina at Chapel Hill, Chapel Hill, NC 27599; bDepartment of Biochemistry & Biophysics, University of North Carolina at Chapel Hill, Chapel Hill, NC 27599; cLineberger Comprehensive Cancer Center, University of North Carolina at Chapel Hill, Chapel Hill, NC 27599; and dIntegrative Program for Biological and Genome Sciences, University of North Carolina at Chapel Hill, Chapel Hill, NC 27599 Edited by Susan Marqusee, University of California, Berkeley, CA, and approved September 12, 2018 (received for review June 18, 2018) Protein−protein interactions are usually studied in dilute buffered mildly influenced by hard-core repulsions. Berg also predicted solutions with macromolecule concentrations of <10 g/L. In cells, that more-compact dimers are more likely to be stabilized by however, the macromolecule concentration can exceed 300 g/L, hard-core repulsions. Here, we test this idea by changing the resulting in nonspecific interactions between macromolecules. shape of a dimer in a controlled fashion. These interactions can be divided into hard-core steric repulsions Scaled particle theory is based on statistical mechanics. For and “soft” chemical interactions. Here, we test a hypothesis from situations like those investigated here, the theory considers so- scaled particle theory; the influence of hard-core repulsions on a lution nonideality as arising from the presence of cosolutes (13– protein dimer depends on its shape. We tested the idea using a 15). As often applied, the solvent and cosolutes are considered side-by-side dumbbell-shaped dimer and a domain-swapped ellip- hard spheres, which leads to three consequences: Molecules only soidal dimer. Both dimers are variants of the B1 domain of protein interact when they touch, the interaction energy is purely re- G and differ by only three residues. The results from the relatively pulsive, and the energy has a strong distance dependence. The inert synthetic polymer crowding molecules, Ficoll and PEG, sup- inability of billiard balls to interpenetrate is a macroscopic ex- port the hypothesis, indicating that the domain-swapped dimer is ample. Water and cosolute concentration are expressed as vol- stabilized by hard-core repulsions while the side-by-side dimer ume occupancy, Φ, the unitless parameter indicating the fraction shows little to no stabilization. We also show that protein coso- of the volume they occupy. The reversible work (i.e., free energy) lutes, which interact primarily through nonspecific chemical inter- to produce a monomer- and dimer-sized hole in water and in a actions, have the same small effect on both dimers. Our results cosolute-containing solution is calculated, and a thermodynamic suggest that the shape of the protein dimer determines the influ- cycle (16) is used to estimate the change in the free energy ence of hard-core repulsions, providing cells with a mechanism for of dissociation (KD→M) in cosolute solution minus the value − regulating protein protein interactions. in water, i.e., ΔΔG°′D→M, where a positive value indicates stabilization. macromolecular crowding | protein−protein interactions | The theory has several shortcomings, including its spherical scaled particle theory assumptions, neglect of chemical interactions, including solva- tion, and its extreme sensitivity to sphere size and density (17, rotein−protein interactions are essential for maintaining 18). Nevertheless, it has been successfully applied to assess the Pcellular homeostasis (1). Details of their equilibria under effects of crowding-induced hard-core interactions on protein thermodynamically ideal conditions have provided a trove of stability and protein−protein interactions (18–22). For instance, information. Ideality in this sense refers to dilute solutions, its application to protein stability led to the idea that crowding where each monomer contacts only solvent or another monomer, conditions far removed from those in cells where protein− Significance protein interactions evolved. In the cytoplasm, and other cellular compartments and biological fluids, macromolecules can occupy Macromolecular crowding influences protein−protein interac- up to 30% of the volume, and their concentrations often exceed tions via hard-core repulsions and chemical interactions. Scaled 300 g/L (2). particle theory predicts that the effect of hard-core repulsions Protein molecules take part in more-complex interactions depends on the shape of the protein complex, and simple ideas under nonideal conditions. The surrounding macromolecules about chemical interactions predict a dominant role for the influence proteins in two ways, neither of which is significant in chemical qualities of the protein surface. The theory predicts dilute solution. Hard-core repulsions arise from high volume that a collapsed, ellipsoidal, dimer will be stabilized by hard- occupancy, because two molecules cannot occupy the same space core repulsions, whereas a less collapsed, side-by-side, dimer at the same time. This volume exclusion favors the most compact will not. We applied scaled particle theory to two dimers with state of a protein (3). Chemical interactions comprise transient nearly identical surfaces but different shapes. Our results contacts between protein surfaces arising from the diverse support the predictions; crowders that interact primarily chemical landscapes of proteins (4). When repulsive (i.e., like through hard-core repulsions stabilize the ellipsoidal domain- charges), they favor the state that maximizes the distance be- swapped dimer more than the side-by-side dimer, whereas tween charges, adding to the hard-core repulsions and stabilizing crowders that interact via chemical interactions have the same the native state. Attractive chemical interactions (e.g., opposite effect on both dimers. charges, hydrogen bonds) are destabilizing. We are beginning to understand how hard-core repulsions and chemical interactions Author contributions: A.J.G., G.B.Y., and G.J.P. designed research; A.J.G., G.M.P.G., and affect protein stability (5), but there are few studies about S.L.S. performed research; A.J.G. contributed new reagents/analytic tools; A.J.G., CHEMISTRY crowding effects on protein−protein interactions (6–9). G.M.P.G., S.L.S., and G.J.P. analyzed data; and A.J.G., G.M.P.G., S.L.S., G.B.Y., and G.J.P. Given the existential roles of both protein−protein interac- wrote the paper. tions and crowding in biology (10), we are undertaking efforts to The authors declare no conflict of interest. determine the effect of crowding on the simplest of protein This article is a PNAS Direct Submission. complexes, homodimers. Our first endeavor, which involved a Published under the PNAS license. side-by-side homodimer, highlighted chemical interactions and 1To whom correspondence should be addressed. Email: [email protected]. showed a small contribution from hard-core repulsions (11, 12). This article contains supporting information online at www.pnas.org/lookup/suppl/doi:10. These results were predicted by Berg (12), who used scaled 1073/pnas.1810054115/-/DCSupplemental. BIOPHYSICS AND particle theory to suggest that side-by-side dimers would be only Published online October 9, 2018. COMPUTATIONAL BIOLOGY www.pnas.org/cgi/doi/10.1073/pnas.1810054115 PNAS | October 23, 2018 | vol. 115 | no. 43 | 10965–10970 Downloaded by guest on October 9, 2021 The A34F variant (Fig. 2) forms a dimer reminiscent of kissing spheres (Figs. 1 and 3) that does not require a large conforma- tional change (26, 28). Dimerization occurs because the Phe-34 side chain becomes part of the hydrophobic core, displacing the Tyr-33 side chain at the surface and causing the C-terminal end of the sole helix to unfold, forming a pocket for the Tyr-33 side chain of the other monomer. The dimer is also stabilized by hydrogen bonds along the now adjacent β-sheets. The L5V;F30V;Y33F;A34F variant forms a more compact domain-swapped dimer (Fig. 2) whose monomer is a partially folded, molten-globule-like species (25, 27) (SI Appendix, Fig. S1) that undergoes refolding to form the dimer (Fig. 3). Swapping the turn between β-strand three and β-strand four results in inter- changed secondary structural units with concomitant formation of several interprotein interactions. The volumes, solvent accessible surface areas, changes in solvent-accessible surface area (SASA), and measured free energies of dissociation are shown in Fig. 3. The properties of protein surfaces determine the chemical ΔΔ o′ Fig. 1. Scaled particle theory-derived values of G D→M as a function of interactions with cosolutes (29–33). An advantageous aspect of eccentricity, L, at 10 values of L (solid symbols) of the indicated radii and the GB1 system is that the interactions are similar for both di- Φ volume occupancies, . As predicted by Berg (12), the more compact mers because their primary structures, and hence their surfaces, spherical, but not the side-by-side, dimer is stabilized. Shaded areas indicate are highly similar (Fig. 3). However, the change in SASA for the the estimated eccentricities of the GB1 dimers. The 4.1-Å species represents sucrose, and the 35-Å species represents BSA. domain-swapped dimer does not reflect its interface area, be- cause the peptide chains penetrate each other. In summary, the GB1 system is well suited for studying crowding effects on pro- effects comprise both hard-core repulsions and attractive or re- tein complex shape, because the monomers are not far removed pulsive chemical interactions (16, 23). from spheres, and the dimers have different shapes but similar We use the implementation of scaled particle theory (13) surfaces. described by Berg (12) to assess crowding effects. The monomers are assumed to be spheres. The dimers have twice the volume of Results the monomer, but their shapes, quantified as the eccentricity, L, Scaled Particle Theory Predictions. Positive values of ΔΔG°′D→M is varied (Fig. 1).
Details
-
File Typepdf
-
Upload Time-
-
Content LanguagesEnglish
-
Upload UserAnonymous/Not logged-in
-
File Pages6 Page
-
File Size-