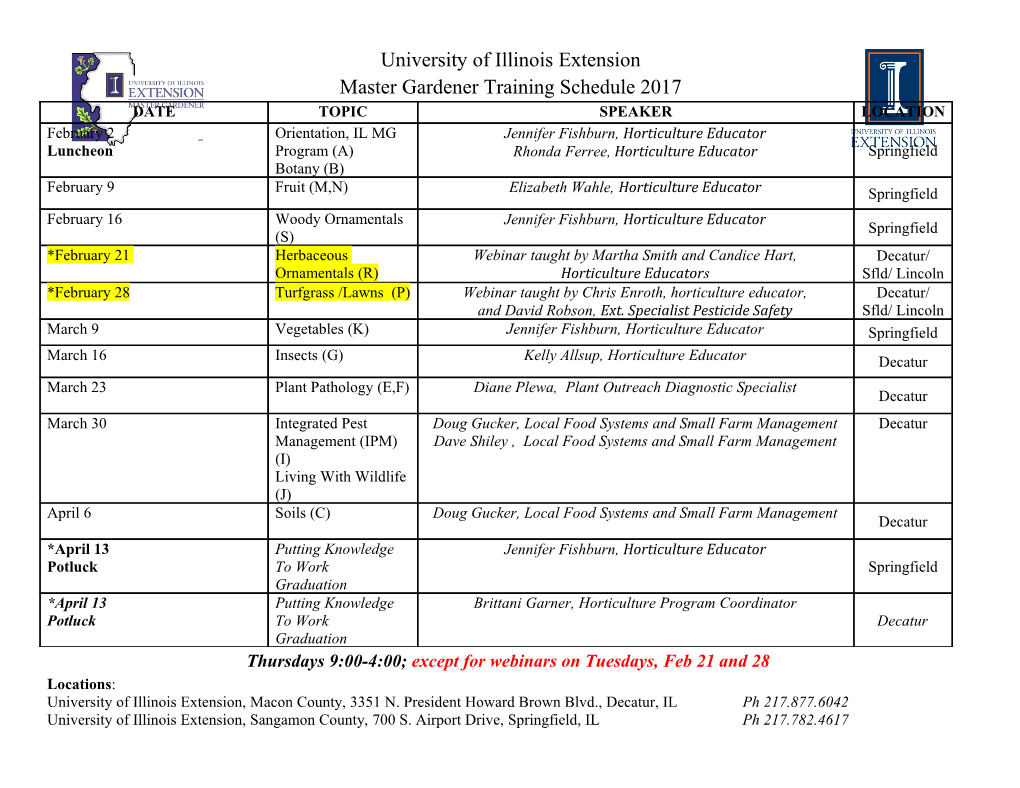
VARIATIONS IN BACTERIAL ADENOSINE TRIPHOSPHATE VALUES DUE TO GENUS AND ENVIRONMENTAL CONDITIONS by Ruann Knox Hampson Thesis submitted to the Faculty of the Virginia Polytechnic Institute and State University in partial fulfillment of the requirements for the degree of Master of Science in Food Science and Technology APPROVED: M. D. Pierson, Chairman J. K. Palmer A. A. Yousten December, 1986 Blacksburg, Virginia VARIATIONS IN BACTERIAL ADENOSINE TRIPOSPHATE VALUES DUE TO GENUS AND ENVIRONMENTAL CONDITIONS by Ruann Knox Hampson Committee Chairman: Merle D. Pierson Food Science and Technology (ABSTRACT) Variations in ATP content in three ground beef spoilage bacteria, Lactobacillus brevis, Lactobacillus jensenii, and Pseudomonas sp. were investigated using the bioluminescence (luciferin-luciferase) assay. Environmental factors (temperature, atmosphere, pH, aeration, and phase of growth), as well as differences among genera and species, were studied in relation to their effect on cellular ATP. Variations for each of the environmental factors and bacteria were shown statistically to be significantly different at the 0.05 level. The mean ATP/cell for each of the bacteria was 2.71 fg/cell (1..:_ brevis), 2.20 fg/cell (1..:_ jensenii), and 1.36 fg/cell (Pseudomonas sp.). For all three bacteria, ATP/cell was lower and more stable throughout the culture's growth cycle at 3°c or in N2 . In general, ATP/cell increases from a lowest value in lag phase to a highest value in stationary phase. The effect of sonication on ATP/cell was tested for each bacterium at one set of factors. Sonication studies showed that L. brevis cells were clumping, especially in aged cultures. After sonication, ATP/cell remained relatively constant from lag through stationary phase. L. jensenii showed no signs of clumping and ATP/cell increased as the culture aged. Sonication had a lethal effect on the Pseudomonas. Thus the ATP/cell for Pseudomonas increased dramatically as the culture aged. Guidelines concerning temperature, assumed ATP content for major contaminants, and sample handling must be followed in order to use the bioluminescence assay to estimate biomass in foods. Acknowledgements This research project was funded by Packard Instruments, a division of United Technologies, Chicago, Illinois. I would like to thank their members for their financial support and suggestions. I also would like to thank the members of my Graduate Committee, Dr. Merle D. Pierson, Dr. James K. Palmer, and Dr. Alan A. Yousten, for their guidance and suggestions. Many other members of the faculty and staff of the Food Science and Technology Department also deserve my thanks for their assistance. I would like to give special thanks to John Chandler for his technical assistance. Special thanks go to the Food Microbiology group -- Joan Hedrick, Jeff Rhodehamel, Fred Cook, Rukma Reddy, Adnan Ismaiel, Rebecca Rorrer, and Marty Bucknavage -- for their support and encouragement. To my husband, parents and friends, I give my love and thank them for all their emotional support (especially at three am), patience, and good humor. iv Table of Contents page Abstract . ii Acknowledgements iv I. Introduction .1 II. Literature Review. .3 A. Biolurninescence. 3 1. Mechanism. .3 2. Factors Affecting Light Emission Color .5 3. Luciferase Specificity .6 4. Inhibitors .8 5. Applications of the Biolurninescence Assay. 10 B. Adenosine Triphosphate .. 11 1. Biological Importance. 11 2. Biochemical Approach to ATP Content Determinations 13 3. ATP per Cell in Bacteria . 16 C. Bacterial Flora of Ground Beef 21 1. Natural Flora of Ground Beef 21 2. Spoilage in Aerobically Packaged Ground Beef 22 3. Spoilage in Vacuum Packaged Ground Beef. 23 III. Materials and Methods .. 25 A. Ground Beef Spoilage Organisms 25 1. Isolation. 25 2. Identification 26 B. ATP Determination. 26 v 1. Equipment. 26 2. Reagent Preparation. 27 a. Water. 27 b. Luciferin - Luciferase 27 c. Buffer 27 d. Extraction Reagent 28 e. Stock ATP Standard 28 f. ATP Standards. 28 g. Internal Standard. 28 h. Stock Phosphate Buffer 28 i. Butterfield's Phosphate Buffer 29 3. Instrument Program 29 4. ATP Calibration. 29 5. ATP Determinations of Unknown Samples. 30 c. Media Preparation. 30 1. Growth Media 30 2. Enumeration Media. 30 D. Experimental Protocol. 31 1. Factors Examined 31 2. Preliminary Growth Curve 31 3. Sampling Growth Curve. 33 4. Growth Curves with Sonication. 33 E. Statistical Analysis 34 IV. Results and Discussion 35 A. Spoilage Isolates. 35 vi B. Preliminary Growth Curve . 37 C. Variations in ATP Content in Bacteria. 50 1. Statistical Analysis 50 2. Effect of Bacterium, Temperature, Growth Atmosphere, pH, and Aeration on ATP Content 52 D. Sonication Experiments 67 v. Summary and Conclusions. 80 Literature Cited 85 Vitae ... .... 94 vii I Introduction Millions of dollars are spent each day by the American public on fresh and processed meat and vegetable products. The food industry has the responsibility of supplying high quality food to the consumer that is nutritious as well as chemically and microbiologically safe. The food industry is regulated by federal, state, and local governments to operate under good manufacturing practices. More important than legal restrictions to the food industry should be the trust of the public in their products. For without public trust, the industry cannot survive in the marketplace. Ground beef has become a common component of the American diet. By its very nature, ground beef has a microbial content of 105 or higher. Examinations of ground beef for microbial load are routinely made by the food industry's quality control personnel. However, the techniques now used necessitate an undesirably long interim (24 to 72 hours incubation time) between sampling and the test's completion. Examinations of this type are both laborious and costly. For years the food industry has worked to develop techniques for the detection and enumeration of foodborne microorganisms that are rapid, reliable, and reproducible. Bioluminometry shows promise of being a rapid method for estimation of bacterial loads in foods. In this method bacterial numbers are estimated by the measurement of adenosine triphosphate (ATP). In the bioluminescence assay luciferase reacts with luciferin and ATP in the presence of oxygen to produce light. This light can be measured and the 1 2 quantity used to determine the amount of ATP present. From this number the bacterial load of the food sample can be determined within an hour. But how reliably can ATP be used to estimate bacterial numbers? Karl (53) stated four assumptions that are made when ATP is used as an estimator of biomass. The first is that ATP could be easily extracted from cells and then measured. The second is that all living organisms contain ATP. Third, ATP is not associated with dead cells. The final assumption is that there is a fairly constant ratio of ATP to total cell carbon for all bacteria. It is this last assumption that has been the least studied. It is the purpose of this study to examine how bacterial ATP content varies due to environmental factors (temperature, pH, atmosphere, aeration, and phase of growth) within as well as between genera and species. II Literature Review A. Bioluminescence 1. Mechanism Bioluminescence is the production of light by an enzyme catalyzed chemical reaction. Harvey (41) reviewed the various types of known luminescent organisms. Included in these are certain bacteria, fungi, sponges, snails, squid, fish, and fireflies. Firefly bioluminescence is the most studied bioluminescence system. McElroy, Seliger, and White (71) determined the reaction mechanism of firefly bioluminescence. The reaction sequence can be swrunarized as follows (68): 1. LUCIFERIN +Mg-ATP + LUCIFERASE -> LUCIFERASE-LUCIFERIN-AMP + PP. 1 2. LUCIFERASE-LUCIF~RIN-AMP + o2 -> LUCIFERASE-OXYLUCIFERIN * + co2 + AMP 3. LUCIFERASE-OXYLUCIFERIN* => LUCIFERASE + OXYLUCIFERIN + LIGHT Luciferin must be present as the D(-) isomer in order to be biologically active (Fig. 1). When luciferin, luciferase, and oxygen are present in excess, ATP becomes the limiting reagent. Thus, the amount of light produced is directly related to the amount of ATP present. For this reason bioluminescence can be used as a sensitive method of assaying ATP 3 4 OXYLUCIFERIN 0 1-) LUCIFERIN Figure 1 Chemical structures of D-(-)-luciferin, the active isomer of luciferin, and oxyluciferin, the oxidized product of the luciferin-luciferase reaction. 5 content (68). If ATP is present in excess, ATP and luciferin-AMP competitively inhibit Mg-ATP on the active site of luciferase (59,66). High pyrophosphate levels also inhibit light production (59). Care must be taken to avoid these types of inhibition in the ATP assay. Luciferase is a protein composed of two 50,000 dalton subunits (66). Denburg, Lee, and McElroy (23) showed that two luciferin binding sites exist on luciferase. Each subunit has a luciferin and ATP binding site but there is only one site on the enzyme for Mg-ATP, the active form of the substrate (66,69). From all information available, McElroy and DeLuca (66) hypothesized that only one subunit is enzymatically active. Once Mg-ATP binds to this active site, conformational changes occur in the structure of luciferase (20). 2. Factors Affecting Light Emission Color McElroy, White, and Seliger (71) showed that for Photinus pyralis, the luciferin-luciferase reaction with ATP emits a yellow-green light (562 nm) when the reaction takes place at a neutral or alkaline pH (optimum pH is 7.75 (97)). The peak emission wavelength varies according to the species of firefly used for luciferase isolation (6,70,86). If the reaction takes place under non-optimal conditions, a red light (614 nm) (which has a lower quantum yield than that of the yellow-green light) is emitted. This red shift occurs as the temperature rises above 23°C (71). The stereochemistry of nucleotide attachment (67) as well as the presence of urea, zn2+, Cd2+, or Hg2+ (71) can also cause this shift to red light.
Details
-
File Typepdf
-
Upload Time-
-
Content LanguagesEnglish
-
Upload UserAnonymous/Not logged-in
-
File Pages101 Page
-
File Size-