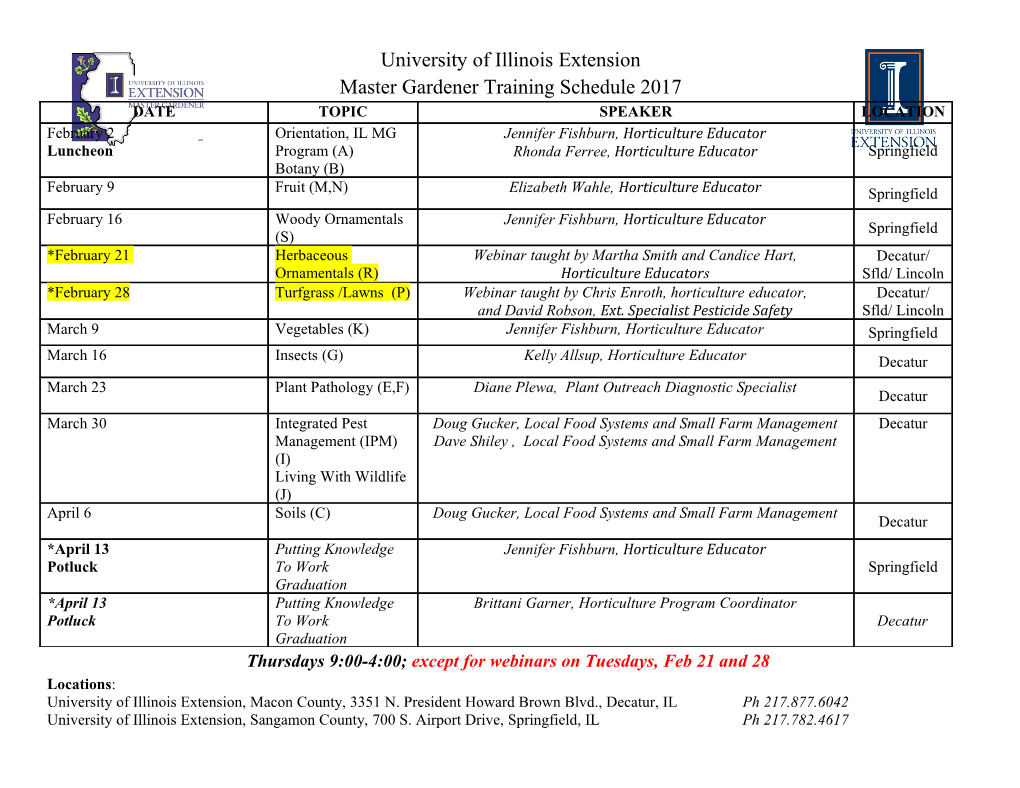
Self-Organising Autocatalysis Nathaniel Virgo1;3, Simon McGregor2;4 and Takashi Ikegami1;5 1Ikegami Laboratory, University of Tokyo, Japan 2University of Sussex, UK [email protected] [email protected] [email protected] Abstract When held out of equilibrium, this chemistry formed mod- erately large and complex autocatalytic cycles. (That is, Life on Earth must originally have arisen from abiotic chem- moderately large sets of species that are collectively capa- istry. Since the details of this chemistry are unknown, we ble of exponential growth.) Unlike many previous studies, wish to understand, in general, which types of chemistry can lead to complex, life-like behaviour. Our recent work this chemistry did not include any a priori notion of catal- has shown that the inclusion of thermodynamic principles ysis. Instead, catalytic effects emerged as dynamical prop- in simple artificial chemistry models can result in the self- erties of the reaction network. The system was allowed to organisation of autocatalytic cycles. In this paper we present dynamically “choose” the direction of its reactions accord- some new insights into why this happens. Our model is ing to thermodynamic principles, and it was this property given a more mathematical treatment, allowing us to better understand the assumptions that lead to this phenomenon. that allowed it to self-organise into autocatalytic cycles. The simplest type of autocatalytic cycle results in exponential This phenomenon of self-organising autocatalysis hap- growth. Through dynamical simulation we demonstrate that pens under some circumstances but not others. In order to when these simple first-order cycles are prevented from form- ing, the system achieves super-exponential growth through fully understand the result we must investigate which classes more complex, higher-order autocatalytic cycles. This leads of reaction networks will lead to autocatalysis and which to non-linear phenomena such as oscillations and bistability, will not. Moreover, the resulting cycles can be more or the latter of which is of particular interest regarding the ori- less complex, and we wish to understand what factors af- gins of life. fect this. A comprehensive answer to this question would be a great help in understanding the origins of life. If we Introduction know which types of chemical system are likely to lead to complex, metabolism-like reactions then it will give us clues How can abiotic chemistry give rise to phenomena such as about what to look for in identifying the types of abiotic replication, metabolism and evolution? This question is at chemistry from which life eventually arose. the core of the origins of life as a field of inquiry, and there are many approaches to it. One such methodology is ar- In this paper we continue this project. We present some tificial chemistry, the study of more-or-less abstract artifi- new insights into why autocatalysis occurs in far-from- cial chemical reaction networks and their emergent dynam- equilibrium systems, and into what is needed for this to hap- ical properties. This field can be traced back to the work pen. As with all non-equilibrium phenomena, the autocatal- of Fontana and Buss (1994) and Kauffman (1986). More ysis in our systems is caused by the tendency of energy (and recently it has been used to show that evolution can occur other conserved currents) to “flow downhill” from more to without the need for template replication (Vasas et al., 2012), less constrained states, i.e. from low to high entropy. Infor- and to investigate the mechanism behind HCN polymerisa- mally, it seems that nature likes to take the “path of least tion, a prebiotically-relevant reaction that is too complex to resistance” in order to achieve this. If the easiest path is a model with traditional approaches (Andersen et al., 2013). simple, direct chemical reaction then no complex behaviour In these models, thermodynamic principles play at best will be observed. Without a “direct” pathway from initial a minor role. However, the nature of living organisms as conditions to equilibrium, autocatalytic pathways tend to be far-from-equilibrium structures is fundamental to our under- observed. Blocking the simplest type of autocatalytic cycle standing of biology at the chemical level. Our work aims to results in the formation of more complex cycles, giving rise show that new insights can be gained by putting thermody- to non-linear phenomena such as bistability and oscillations. namics at the heart of artificial chemistry approaches. Autocatalytic cycles can only emerge if they are possi- Last year we presented a simple yet thermodynamically ble within the reaction network. However, if the direction reasonable artificial chemistry (Virgo and Ikegami, 2013). in which reactions occur is not fixed a priori then even the ALIFE 14: Proceedings of the Fourteenth International Conference on the Synthesis and Simulation of Living Systems simplest reaction schemes contain many autocatalytic sub- rates as the net rate of the bidirectional reaction, A+B )−*− C. networks. The dynamics must be such that, in the absence of any The results we present are very robust. With one minor energy sources, the system will eventually reach a state of exception (the oscillatory behaviour shown in Figure 3) they detailed balance in which the net rate of every reaction is require no parameter tuning at all. Moreover, our mathe- zero. That is, every forward reaction must be balanced, pair- matical arguments are applicable to a much broader class of wise, by its corresponding reverse reaction. (This point is chemical systems than the specific models we present. Thus, of fundamental importance. In a general artificial chemistry although our model is relatively simple and abstract, we ex- a dynamical equilibrium can be formed by a cycle, such as pect that in future work similar results will be found in much A −! B; B −! C; C −! A. In a thermodynamic system with more realistic scenarios. no driven reactions this is a physical impossibility.) Every net reaction must always proceed in the direction that takes Thermodynamics in Artificial Chemistry the system closer to this equilibrium state. Thus, no reaction A glance at a chemistry text book will reveal a large number can occur at a non-zero net rate unless the system is either of formulae that appear to be largely empirical in nature, and in a transient state or is being driven by an external power this can give the impression that chemical thermodynamics source. is something very contingent upon the details of molecular In our model we use a simple implementation of these interactions. Perhaps because of this, there is a tendency dynamics, known as mass action kinetics. Essentially this in artificial chemistry to “abstract away” thermodynamics, corresponds to a model of a well-mixed reactor in which choosing a set of reactions arbitrarily, without regard to en- molecules collide with each other at random. When two ergetic considerations. This may be justified by saying that molecules collide they react with a probability determined some of the reactions are driven by an energy source that is by the “rate constant” of the reaction. Thermodynamics external to the system and is not explicitly modelled. This puts constraints on the values of the rate constants; these methodology dates back to the origins of artificial chemistry constraints are satisfied by the dynamics described below. in the work of Fontana and Buss (1994). (See Kondepudi and Prigogine (1998) for details of the for- Such an abstraction is of course perfectly valid, but we malism, and Virgo and Ikegami (2013) for its application to hope to show that important insights can be gained if we models of the type described below.) choose not to make it. The dynamics of a system where there are only a few energy sources and the majority of reac- The A-polymerisation model tions must “flow downhill” are quite different from those in When implemented in a thermodynamically plausible way, which the reactions proceed in arbitrarily-chosen directions. even very simple reaction schemes can give rise to interest- Moreover, the flow of free energy through the network can ing behaviour. Our model consists of unary strings A, AA, be tracked, giving us insights into its dynamics that would AAA etc., which we write as A ; A ; A ;::: . In principle be missing if the energetics were not modelled. 1 2 3 these are countably infinite, but we impose a limit for com- Although chemical thermodynamics might appear to be a putational reasons. These species can be thought of as (non- rather empirical set of results, its core principle (the second oriented) polymers, with A (or simply A) representing a law) is an extremely fundamental property of physical sys- 1 monomer, and A a polymer (or oligomer) of length i. All tems. Essentially, it boils down to a requirement that for a i reactions are of the form system with no external supply of energy, there must exist a global Lyapunov function, which may be called the entropy A + A )−−−−* A : (1) or the free energy, depending on whether the system is con- i j i + j nected to a heat bath. The existence of such a function is de- That is, two oligomers may join together into a longer one, rived from statistical mechanics, and is a consequence of the or an oligomer may split into two smaller ones. Reactions time-reversibility of physics on the microscopic level. The of this form conserve the total number of A monomers. We details of this formalism are beyond the scope of this paper take the forward and reverse reaction rates to be constants k and may be found, for example, in Kondepudi and Prigogine s and k , (for “synthesis” and “decomposition”) with the same (1998).
Details
-
File Typepdf
-
Upload Time-
-
Content LanguagesEnglish
-
Upload UserAnonymous/Not logged-in
-
File Pages8 Page
-
File Size-