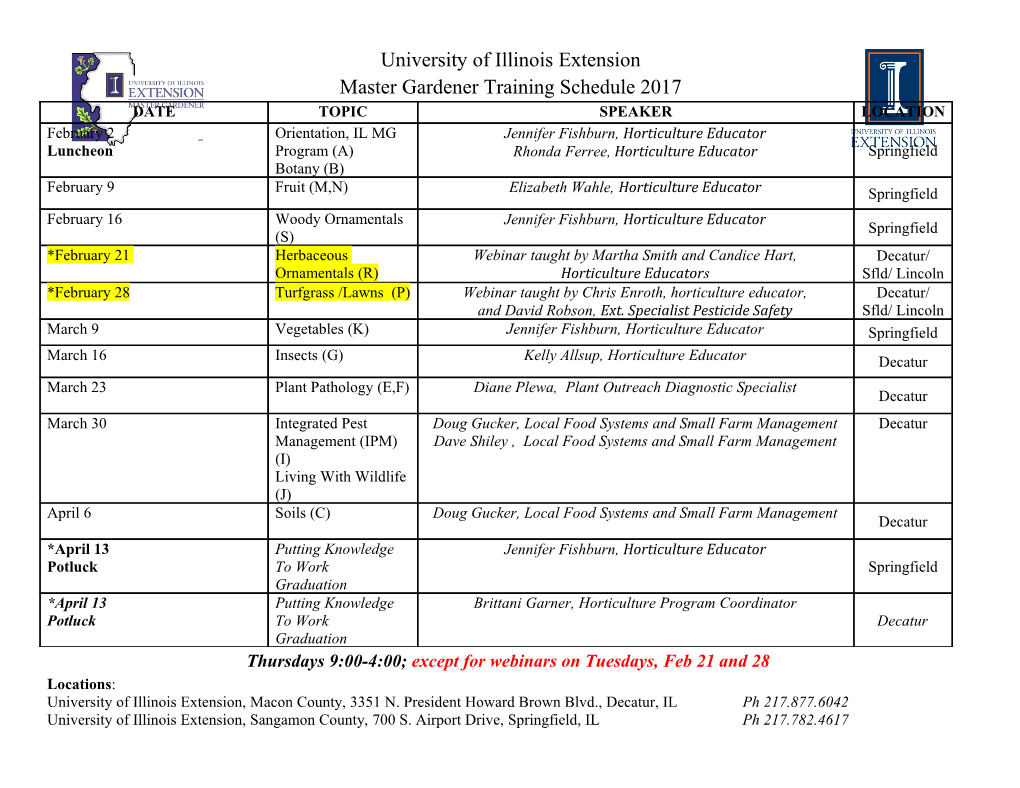
Meteoritics & Planetary Science 38, Nr 4, 515–527(2003) Abstract available online at http://meteoritics.org Experimental and petrological constraints on lunar differentiation from the Apollo 15 green picritic glasses Linda T. ELKINS-TANTON,1* Nilanjan CHATTERJEE,2 and Timothy L. GROVE2 1Department of Geological Sciences, Brown University, Providence, Rhode Island, 02912, USA 2Department of Earth, Atmospheric and Planetary Sciences, Massachusetts Institute of Technology, Cambridge, Massachusetts 02139, USA *Corresponding author: [email protected] (Received 15 July 2002; revision accepted 23 September 2002) Abstract–Phase equilibrium experiments on the most magnesian Apollo 15C green picritic glass composition indicate a multiple saturation point with olivine and orthopyroxene at 1520°C and 1.3 GPa (about 260 km depth in the moon). This composition has the highest Mg# of any lunar picritic glass and the shallowest multiple saturation point. Experiments on an Apollo 15A composition indicate a multiple saturation point with olivine and orthopyroxene at 1520°C and 2.2 GPa (about 440 km depth in the moon). The importance of the distinctive compositional trends of the Apollo 15 groups A, B, and C picritic glasses merits the reanalysis of NASA slide 15426,72 with modern electron microprobe techniques. We confirm the compositional trends reported by Delano (1979, 1986) in the major element oxides SiO2, TiO2, Al2O3, Cr2O3, FeO, MnO, MgO, and CaO, and we also obtained data for the trace elements P2O5, K2O, Na2O, NiO, S, Cu, Cl, Zn, and F. Petrogenetic modeling demonstrates that the Apollo 15 A-B-C glass trends could not have been formed by fractional crystallization or any continuous assimilation/fractional crystallization (AFC) process. The B and C glass compositional trends could not have been formed by batch or incremental melting of an olivine + orthopyroxene source or any other homogeneous source, though the A glasses may have been formed by congruent melting over a small pressure range at depth. The B compositional trend is well modeled by starting with an intermediate A composition and assimilating a shallower, melted cumulate, and the C compositional trend is well modeled by a second assimilation event. The assimilation process envisioned is one in which heat and mass transfer were separated in space and time. In an initial intrusive event, a picritic magma crystallized and provided heat to melt magma ocean cumulates. In a later replenishment event, the picritic magma incrementally mixed with the melted cumulate (creating the compositional trends in the green glass data set), ascended to the lunar surface, and erupted as a fire fountain. A barometer created from multiple saturation points provides a depth estimate of other glasses in the A-B-C trend and of the depths of assimilation. This barometer demonstrates that the Apollo 15 A-B-C trend originated over a depth range of ~460 km to ~260 km within the moon. INTRODUCTION on the early thermal history and chemical structure of the moon. These boundary conditions apply from about 4.5 to 4.3 This work focuses on the compositional variability Ga, when the magma ocean cooled and solidified to create displayed in Apollo 15 green glasses, groups A, B, and C, as differentiated chemical reservoirs, and also from 3.3 to 3.5 defined by Delano (1979). The Apollo 15C green glass has Ga, when the magmas melted from the differentiated the highest Mg# of any picritic glass yet found on the moon reservoirs and erupted (e.g., Delano 1986; Papike et al. 1998). (Mg# = molar [Mg/(Mg + Fe)] × 100). The compositional Because little is known about the early evolution of the trends of the Apollo 15 A-B-C green glasses are distinctive interior of the moon, there are several potential models for and span a broad range of compositions. They vary in Mg# the processes that formed the parental green glasses, the from 60.6 to 67.4 (MgO from 17.1 to 18.6 wt%), in SiO2 from progenitors of the compositional trends seen in each picritic 45.5 to 48.5 wt%, and in FeO from 20 to 16 wt%. The glass group (Fig. 1), and the compositional trends processes that created these trends place boundary conditions themselves. 515 © Meteoritical Society, 2003. Printed in USA. 516 L. T. Elkins Tanton et al. coefficients, and the fluorescence corrections from Reed, was used to reduce the data and obtain quantitative analyses. A materials balance calculation was used to estimate phase proportions and to determine whether Fe had been lost from the experimental charge. The results are reported in Table 2 as the R2, the sum of squared residuals, and as phase proportions. Experiments on a Synthetic Analogue of an Apollo 15A Composition The near-liquidus phase relations of a synthetic analogue of an Apollo 15A green glass (Delano 1986; Table 1) were Fig. 1. Possible formation processes for the Apollo 15 A-B-C picritic glasses discussed in this paper. determined in experiments from 1 atm to 2.5 GPa and at temperatures from 1400°C to 1570°C at SUNY Stony Brook In the discussion section below, we critique the in 1978 (see also Grove and Vaniman [1978], for phase application of these models to the Apollo 15 A, B, and C relations at 1 atm and experimental techniques). The trends. Based on compositions and experimentally crystalline phases were analyzed at SUNY Stony Brook on determined multiple saturation points, a number of models an ARL-EMX-SM microprobe using the data correction can be ruled out without making any assumptions about the procedures of Bence and Albee (1968) with the modifications phases present in the lunar mantle. of Albee and Ray (1970). A materials balance calculation was used to estimate phase proportions and to determine EXPERIMENTAL AND ANALYTIC METHODS whether Fe had been lost from the experimental charge. These results are reported in Table 3 as the R2, the sum of Experiments on a Synthetic Analogue of an Apollo 15C squared residuals. Composition Reanalysis of Green Glasses of Sample 15426,72 The near-liquidus phase relations of a synthetic analogue of the highest Mg# Apollo 15C green glass (Delano 1986) The glass beads in slide 15426,72 were analyzed using were determined in experiments from 1 atm to 2.0 GPa the MIT JEOL-JXA-733 Superprobe. Following normal (corresponding to near surface to about 400 km depth in the probe calibration procedures, analyses were further calibrated moon) and at temperatures from 1350°C to 1550°C. The among sessions by comparing the repeated analysis of two specific green glass chosen defines the high Mg# end of the glass beads, designated by Delano as 31A and 131A. Each Apollo green glass trends, and, therefore, of all the lunar glass bead was analyzed five times with a 10 micron beam picritic glasses (Table 1). Piston-cylinder experiments were spot size, using a 10 nA beam current and an accelerating carried out as described in Elkins et al. (2000), with a voltage of 15 kV, for oxide components SiO2, TiO2, Al2O3, difference in assembly. By placing the experimental charge in Cr2O3, FeO, MnO, MgO, CaO, Na2O, K2O, and P2O5. Only a graphite capsule without a Pt sleeve, we reduced cracking in glass analyses with totals between 99.00 and 101.00 oxide the capsule and iron loss in these low-viscosity compositions. wt% were used. During a separate analysis session, we To obtain crystal-free glass, the experiments were quenched measured the trace elements Ni, S, Cl, Cu, Zn, and F. Again, by shutting off the power to the furnace and simultaneously five separate analyses of each bead were done, with a 10 dropping the pressure on the experiment to 0.8 GPa. Using micron spot size but a 200 nA beam current and counting this technique resulted in analyzable glass unmodified by times up to 300 seconds. The data were reduced and corrected disequilibrium pyroxene crystals in about 95% of the as described for the Apollo 15C experiments above. experiments, while without this technique, only about 25% of the experiments contained analyzable glass. The experimental EXPERIMENTAL AND ANALYTIC RESULTS charges were mounted in 1′′ epoxy disks, polished, and carbon-coated. The crystalline phases were analyzed at MIT The Apollo 15C Composition on a JEOL-JXA-733 Superprobe at an accelerating voltage of 15 kV, beam current of 10 nA, and beam diameter of 1 The phase diagram for the Apollo 15C glass is shown in micron. Glasses in the experimental charges were analyzed Fig. 2. The 18 experiments locate a multiple saturation point with a beam diameter of 10 microns. The CITZAF correction with olivine and orthopyroxene on the liquidus at 1.3 GPa package of Armstrong (1995), using the atomic number (~260 km lunar depth) at 1520°C. Olivine is the liquidus correction from Duncumb and Reed, the absorption phase from 1 atm pressure and ~1380°C to the multiple corrections with Heinrich’s tabulation of mass-absorption saturation point at 1.3 GPa and 1520°C. Above the multiple Experimental and petrological constraints on lunar differentiation 517 Table 1. Experimental starting materials. SiO2 TiO2 Al2O3 Cr2O3 FeO MnO MgO CaO Na2OK2O Total Mg# A15A 46.01 0.42 7.93 0.46 18.88 0.24 17.58 8.48 0.00 0.00 100.00 62.39 A15C 48.29 0.23 7.77 0.55 16.12 0.19 18.27 8.59 0.00 0.00 100.00 66.89 Fig. 3. Phase diagram of Apollo 15A green glass synthetic analogue. The experiment numbers are given next to the symbols. Fig. 2. Phase diagram of Apollo 15C green glass synthetic analogue. The experiment numbers are given next to the symbols.
Details
-
File Typepdf
-
Upload Time-
-
Content LanguagesEnglish
-
Upload UserAnonymous/Not logged-in
-
File Pages13 Page
-
File Size-