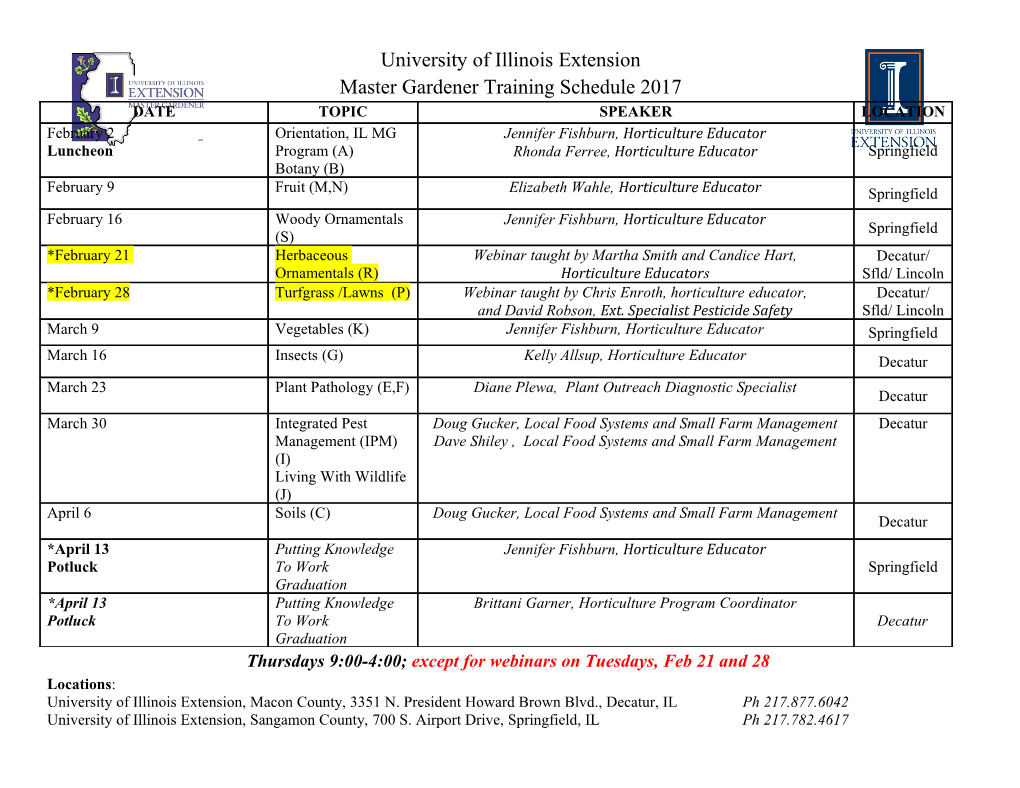
AMPLITUDE INVERSION OF FAST AND SLOW CONVERTED WAVES FOR FRACTURE CHARACTERIZATION OF THE MONTNEY FORMATION IN POUCE COUPE FIELD, ALBERTA, CANADA by Tyler L. MacFarlane c Copyright by Tyler L. MacFarlane, 2014 All Rights Reserved A thesis submitted to the Faculty and the Board of Trustees of the Colorado School of Mines in partial fulfillment of the requirements for the degree of Master of Science (Geo- physics). Golden, Colorado Date Signed: Tyler L. MacFarlane Signed: Dr. Thomas L. Davis Thesis Advisor Golden, Colorado Date Signed: Dr. Terence K. Young Professor and Head Department of Geophysics ii ABSTRACT The Montney Formation of western Canada is one of the largest economically viable gas resource plays in North America with reserves of 449TCF. As an unconventional tight gas play, the well development costs are high due to the hydraulic stimulations necessary for economic success. The Pouce Coupe research project is a multidisciplinary collaboration between the Reservoir Characterization Project (RCP) and Talisman Energy Inc. with the objective of understanding the reservoir to enable the optimization of well placement and completion design. The work in this thesis focuses on identifying the natural fractures in the reservoir that act as the delivery systems for hydrocarbon flow to the wellbore. Characterization of the Montney Formation at Pouce Coupe is based on time-lapse mul- ticomponent seismic surveys that were acquired before and after the hydraulic stimulation of two horizontal wells. Since shear-wave velocities and amplitudes of the PS-waves are known to be sensitive to near-vertical fractures, I utilize isotropic simultaneous seismic in- versions on azimuthally-sectored PS1 and PS2 data sets to obtain measurements of the fast and slow shear-velocities. Specifically, I analyze two orthogonal azimuths that are parallel and perpendicular to the strike of the dominant fracture system in the field. These volumes are used to approximate the shear-wave splitting parameter (γ(s∗)) that is closely related to crack density. Since crack density has a significant impact on defining the percolation zone, the work presented in this thesis provides information that can be utilized to reduce uncertainty in the reservoirs fracture model. Isotropic AVO inversion of azimuthally limited PS-waves demonstrates sufficient sensi- tivity to detect contrast between the anisotropic elastic properties of the reservoir and is capable of identifying regions with high crack density. This is supported by integration with spinner production logs, hydraulic stimulation history of the field, and microseismic. Results also show significant fracture network heterogeneity that is not typically accounted for in iii engineering-driven development despite a strong link to production. The main value of this work lies in the integration of fracture characterization with preceding RCP theses that defined the geomechanical model and composition of the reservoir at Pouce Coupe. Geophysical attributes that relate to the composition and natural fractures enable a more complete understanding of the reservoir and indicate that a successful well is dependent on both the hydrocarbon storage capacity of the matrix and a large permeable network of natural fractures. iv TABLE OF CONTENTS ABSTRACT . iii LIST OF FIGURES . viii LIST OF TABLES . xiv LIST OF ABBREVIATIONS . xv ACKNOWLEDGMENTS . xvi DEDICATION . xvii CHAPTER 1 INTRODUCTION . 1 1.1 Montney Geology . 3 1.1.1 Stratigraphy . 3 1.1.2 Reservoir Characteristics . 5 1.1.3 Regional Tectonics . 8 1.2 Pouce Coupe data set . 10 1.2.1 Field Development . 10 1.2.2 Time-Lapse Multicomponent Seismic . 13 1.2.3 Microseismic Data . 15 1.3 Previous Pouce Coupe Research . 16 1.4 Research Objective . 24 CHAPTER 2 SEISMIC MODEL OF FRACTURES . 26 2.1 Natural Fracture Background . 27 2.2 Induced/Natural Fracture Interaction . 29 v 2.3 Fracture Compliance Methodology . 30 2.4 Numeric Modeling . 33 2.5 Pouce Coupe Seismic Implications . 39 CHAPTER 3 MULTICOMPONENT SEISMIC DATA PROCESSING . 43 3.1 Fixed Receiver Rotation . 44 3.2 Bin Size and COV Interpolation . 47 3.3 Azimuthal Sectoring . 49 3.4 Processing Conclusions . 52 CHAPTER 4 CONVERTED WAVE SEISMIC INVERSION . 53 4.1 Inversion Theory . 54 4.1.1 Constrained Sparse Spike Inversion . 56 4.2 Available Data and PS Seismic Interpretation . 58 4.3 Low Frequency Model . 61 4.4 PS1 Inversion . 62 4.4.1 Data Conditioning . 66 4.4.2 Well Tie and Wavelet Extraction . 69 4.4.3 Inversion Parameters . 75 4.4.4 Inversion Results and Quality Control . 77 4.5 PS2 Inversion . 80 4.5.1 Data Conditioning . 82 4.5.2 Well Tie and Wavelet Extraction . 82 4.5.3 PS2 Inversion Results and Quality Control . 87 4.6 Inversion Conclusions . 91 vi CHAPTER 5 FRACTURE NETWORK INTERPRETATION AND INTEGRATION . 95 5.1 Shear Velocity Fracture Characterization . 95 5.1.1 Interpretation Limitations . 96 5.1.2 Fracture Map . 97 5.2 Fracture/Production Correlation . 99 5.3 Rock Composition Analysis . 106 5.4 Microseismic . 106 5.5 Comparison to SWS Analysis . 108 CHAPTER 6 CONCLUSIONS AND RECOMMENDATIONS . 112 6.1 Recommendations . 113 REFERENCES CITED . 115 vii LIST OF FIGURES Figure 1.1 Map of the Peace River embayment that bounds the Montney Formation . 4 Figure 1.2 Paleogeographic map of North America during the Early Triassic (245Ma) . 5 Figure 1.3 Montney type log and stratigraphic chart of the Triassic . 6 Figure 1.4 Depositional model of the Montney Formation . 6 Figure 1.5 East-West modeled stratigraphic section of the Montney Formation . 7 Figure 1.6 Map of maximum horizontal stress derived from well bore breakouts. Pouce Coupe field is highlighted by a star . 9 Figure 1.7 Pouce Coupe Field layout and timeline of operations . 11 Figure 1.8 Pouce Coupe Average Daily Gas Production for Montney wells . 13 Figure 1.9 Source and Receiver layout of the Pouce Coupe seismic survey . 14 Figure 1.10 Offset/Azimuth distribution in individual bins of the Pouce Coupe Seismic . 16 Figure 1.11 Microseismic events: a) from the 02/07-07 stimulation recorded by the 08-07 toolstring , b) from the 02/07-07 stimulation recorded by the 09-07 toolstring c) from the 02/02-07 well recorded by the 08-07 toolstring d) from the 02/02-07 well recorded by the 09-07 toolstring . 17 Figure 1.12 Downhole microseismic acquisition geometry . 18 Figure 1.13 Model of SWS . 19 Figure 1.14 Baseline SWS magnitude and PS1 polarization orientation . 21 Figure 1.15 Monitor 1 SWS magnitude and PS1 polarization orientation . 21 Figure 1.16 Monitor 3 SWS magnitude and PS1 polarization orientation . 22 Figure 1.17 Inverted λρ and µρ cross section . 23 viii Figure 1.18 Inverted P-Impedance cross section through well 02/02-07 with geobodies of best clusters . 24 Figure 2.1 Schematic of an orthorhombic model . 26 Figure 2.2 Mohr-Coulomb graphical representation of stress fields producing tensional and shear rock failures . 28 Figure 2.3 Mohr-Coulomb graphical representation of fracture failure produced by increasing pore pressure . 28 Figure 2.4 Simulated hydraulic fracture in the presence of perforations that act as permeable conduits and glass plates that represent cemented natural fractures . 30 Figure 2.5 Schematic model of a medium with multiple sets of parallel fractures . 32 Figure 2.6 Workflow for modeling the seismic response of fractures. 34 Figure 2.7 PS reflection coefficients of the four fracture models associated with the Montney C/D interface along azimuths aligned with the x1 and x2 axes . 38 Figure 2.8 Comparison between anisotropic and isotropic PS reflection coefficient equations used to represent fracture model 3 . 41 Figure 3.1 Previous processing of the Pouce Coupe data set. PS1 is displayed on the left, and PS2 is on the right . 44 Figure 3.2 New AVO compliant processing of the Pouce Coupe data set. PS1 is displayed on the left, and PS2 is on the right . 45 Figure 3.3 Schematic of field components H1/H2, and rotated PS1/PS2 coordinates . 46 Figure 3.4 PS1 orientation as determined by SWS analysis within a time window of 700-900ms . 46 Figure 3.5 Overburden shear-wave splitting for three analysis windows . 48 Figure 3.6 Common offset vector diagram and its relationship to offset and azimuth . 49 Figure 3.7 Old and New AVO compliant PS1 pre-migration stacks . 50 ◦ Figure 3.8 PS1 and PS2 limited azimuth stacks sorted in 10 azimuth sectors from 0◦ to 360◦ ...................................51 ix Figure 3.9 Limited azimuth ranges allowed in PS1 and PS2 volumes . 52 Figure 4.1 Deterministic and stochastic inversion workflow overview . 54 Figure 4.2 Representation of the convolutional model . 55 Figure 4.3 Jason's workflow for the simultaneous CSSI . 57 Figure 4.4 Pouce Coupe basemap with the location of wells 13-12 and 02/07/07 displayed . 59 Figure 4.5 Cross-section of the PS1 seismic with inlayed seismograms from wells 13-12 and 02/07-07. Horizon interpretation is also displayed . 59 Figure 4.6 PS1 time structure map of the a) Triassic b) Montney E c) Belloy . 60 Figure 4.7 Bandwidth of the Pouce Coupe seismic with the lowpass filter applied to the models shown in red and the bandpass filter applied to the inversion result in cyan . 61 Figure 4.8 Inverse distance interpolation technique . 62 Figure 4.9 Cross section of the shear velocity model with a) 30Hz high cut filter applied b) 9Hz high cut filter applied . 63 Figure 4.10 Cross section of the compressional velocity model with a) 30Hz high cut filter applied b) 9Hz high cut filter applied . 64 Figure 4.11 Cross section of the density model with a) 30Hz high cut filter applied b) 9Hz high cut filter applied .
Details
-
File Typepdf
-
Upload Time-
-
Content LanguagesEnglish
-
Upload UserAnonymous/Not logged-in
-
File Pages136 Page
-
File Size-