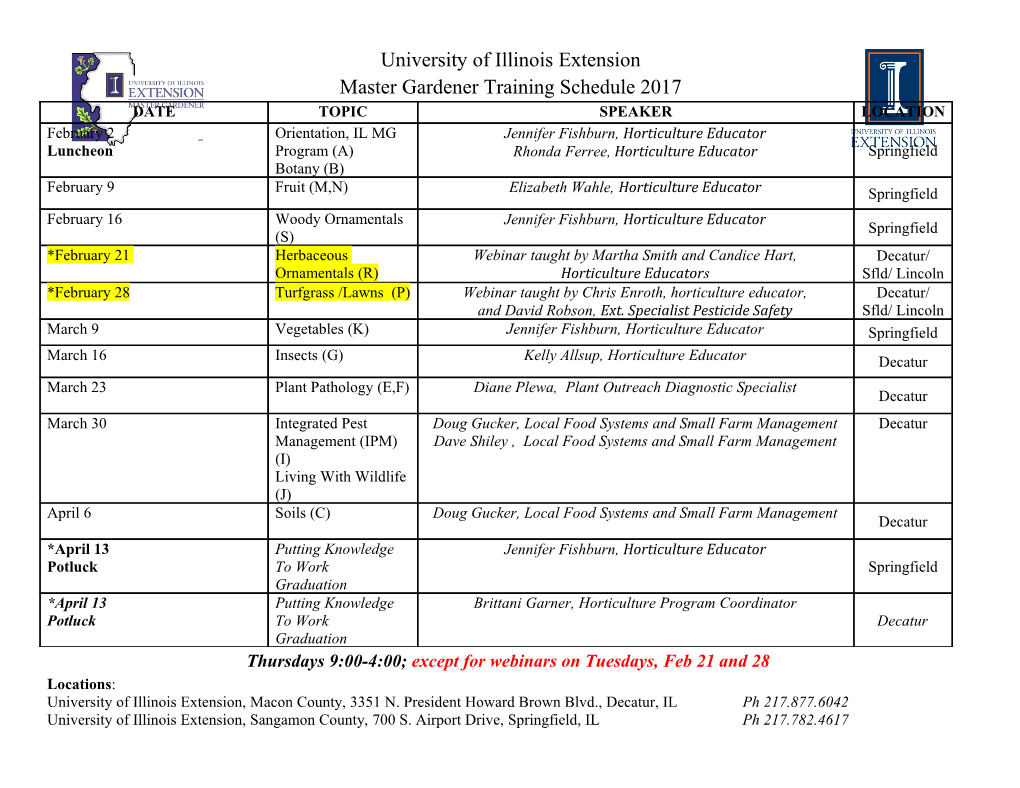
LARGE-PARAMETER ASYMPTOTIC EXPANSIONS FOR THE LEGENDRE AND ALLIED FUNCTIONS GERGO} NEMES AND ADRI B. OLDE DAALHUIS Abstract. Surprisingly, apart from some special cases, simple asymptotic expansions for µ µ the associated Legendre functions Pν (z) and Qν (z) for large degree ν or large order µ are not available in the literature. The main purpose of the present paper is to fill this gap by deriving simple (inverse) factorial expansions for these functions and provide sharp and realistic bounds on their error terms. Analogous results for the Ferrers functions and the closely related Gegenbauer function are also included. In the cases that ν is an integer or 2µ is an odd integer, many of these new expansions terminate and provide finite representations in terms of simple functions. Most of these representations appear to be new. It is well known that the hypergeometric series can be regarded as a large-c asymptotic expansion for the hypergeometric function F (a; b; c; z). We also derive computable bounds for the remainder term of this expansion. To our best knowledge, no such estimates have been given in the literature prior to this paper. 1. Introduction and main results µ In this paper, we derive asymptotic expansions for the associated Legendre functions Pν (z) µ µ µ and Qν (z), and the Ferrers functions Pν (x) and Qν (x) in the case that either the degree ν or the order µ becomes large. These functions are solutions of the associated Legendre differential equation d2w(z) dw(z) µ2 (1.1) (1 z2) 2z + ν(ν + 1) w(z) = 0: − dz2 − dz − 1 z2 − The equation (1.1) occurs in the theory of spherical harmonics, potential theory, quantum me- chanics and in other branches of applied mathematics (see, for example, [4, Ch. 14]). Over the past several decades, a great deal of effort has gone into deriving asymptotic expansions for these functions. Most of these (uniform) expansions are in terms of non-elementary functions (Airy functions, Bessel functions, parabolic cylinder functions, etc.) and often have complicated coefficients (see the end of this section for a short summary). In contrast, the expansions we present in this paper are just (inverse) factorial expansions, with simple, elementary coefficients. In addition, we provide, in Sections2{5, sharp and realistic error bounds for the new expan- sions. Numerical examples demonstrating the sharpness of these bounds and the accuracy of the asymptotic expansions are given in Section 11. Note, however, that unlike their uniform coun- terparts, our expansions break down near the transition points z = 1 of the equation (1.1), except for the large-µ asymptotic expansions for the associated Legendre± functions which require z to be bounded instead. We find it surprising that, apart from some special cases, these simple arXiv:1905.07204v2 [math.CA] 16 Oct 2019 expansions have not been given in the literature before. The reason might be that previous authors were mainly focusing on deriving asymptotic results that are uniform in character, and hence, inevitably posses a rather complicated structure. Some of our results are special cases 2010 Mathematics Subject Classification. 41A60, 33C05, 33C45. Key words and phrases. asymptotic expansions, error bounds, Ferrers functions, Gegenbauer function, hyper- geometric function, Legendre functions. 1 2 G. NEMES AND A. B. OLDE DAALHUIS of the well-known large-c asymptotic expansion of the hypergeometric function F (a; b; c; z), for which we also provide, for the first time, computable error bounds. A common feature of our large-ν expansions below is that they terminate when 2µ equals an odd integer, thereby giving simple, closed-form expressions for the associated Legendre functions and the Ferrers functions. Most of these representations appear to be new. Similarly, the large-µ expansions terminate in the case that ν is an integer and, again, yield simple, finite representations. We begin with the definition of the functions under consideration. The associated Legendre functions of complex degree ν and order µ are defined in terms of the (regularised) hypergeometric function as follows (cf. [4, 14.3(ii)]): x z + 1µ/2 ν + 1; ν 1 z P µ(z) = F − ; − ν z 1 1 µ 2 − − for z C ( ; 1], and 2 n −∞ µ/2 ν+µ+2 ν+µ+1 ! π1=2Γ(ν + µ + 1) z2 1 ; 1 πiµ µ − F 2 2 (1.2) e− Qν (z) = ν+1 ν+µ+1 3 ; 2 2 z ν + 2 z for z C ( ; 1] and ν + µ C Z<0. For analytic continuation to other Riemann sheets, see [4, 14.242 ].n The−∞ Ferrers functions2 ofn complex degree ν and order µ are defined as follows (cf. [4, 14.3(i)x ]): x 1 + xµ/2 ν + 1; ν 1 x (1.3) Pµ(x) = F − ; − ν 1 x 1 µ 2 − − for 1 < x < 1, and − π 1 + xµ/2 ν + 1; ν 1 x Qµ(x) = cos(πµ) F − ; − ν 2 sin(πµ) 1 x 1 µ 2 − − ! Γ(ν + µ + 1) 1 xµ/2 ν + 1; ν 1 x − F − ; − −Γ(ν µ + 1) 1 + x 1 + µ 2 − for 1 < x < 1 and ν + µ C Z<0. When µ is an integer, the right-hand side is replaced by its limiting− value. 2 n We shall also study the closely related Gegenbauer function. The Gegenbauer function of complex degree ν and order λ may be defined as follows (cf. [4, Eq. 14.3.21] and [4, Eq. 14.3.22]): 1 (λ) pπΓ(ν + 2λ) 2 λ (1.4) C (z) = P − 1 (z) ν λ 1 ν+λ Γ(λ)Γ(ν + 1) 2pz2 1 − 2 − 2 − for z C ( ; 1], and 2 n −∞ 1 (λ) pπΓ(ν + 2λ) 2 λ (1.5) C (x) = P − 1 (x) ν λ 1 ν+λ Γ(λ)Γ(ν + 1) 2p1 x2 − 2 − 2 − for 1 < x < 1. − µ Our initial focus is on the large-ν asymptotics. In the case that ν is large, Qν (z) is the recessive solution of differential equation (1.1). Our main tools are the following convenient representations for this function. LARGE-PARAMETER ASYMPTOTICS FOR THE LEGENDRE AND ALLIED FUNCTIONS 3 (1) The associated Legendre function of the second kind can be represented in terms of the modified Bessel function of the second kind as Z + πiµ µ 1 1 ν t cosh ξ (1.6) e− Q (cosh ξ) = t e− K (t sinh ξ)dt; ν Γ(ν µ + 1) µ − 0 provided that ξ > 0 and ν > µ 1 (compare [6, Eq. (6.8.29)] and (1.2)). An immediate consequence of the factor <tν in thej< integrandj − in (1.6) is that when ν is large and positive, the main contribution to this integral comes from large values of t, and< thus we can obtain a large-ν asymptotic expansion by expanding the Bessel function by its well-known large-t asymptotic expansion. In this way it is also straightforward to obtain sharp and realistic error bounds. (2) If combined with a quadratic transformation [4, Eq. 15.8.19] for the hypergeometric func- tion, (1.2) yields the representation r 1 1 ξ ! π 1 + µ, µ e− πiµ µ (ν+ 2 )ξ 2 2 (1.7) e− Qν (cosh ξ) = e− Γ(ν + µ + 1)F 3− ; − ; 2 sinh ξ ν + 2 2 sinh ξ (cf. [7, Eq. (3.2.44)]) in which, again, ν + µ C Z<0 and we take ξ 1 where 2 n 2 D (1.8) = ξ : ξ > 0; ξ < πp ; p > 0: Dp f < j= j g Note that the function cosh ξ is a biholomorphic bijection between 1 and C ( ; 1]. Any hypergeometric function for which a quadratic transformationD existsn can−∞ be expressed in terms of the associated Legendre functions or the Ferrers functions (cf. [4, 15.9(iv)]). Con- sequently, there are several representations in terms of hypergeometric functions.x For large parameter asymptotic approximations of hypergeometric functions see, e.g., [21] and [8]. These results can be used to obtain asymptotic expansions for the associated Legendre functions or the Ferrers functions. In general, the hypergeometric series a; b a; b X1 (a) (b) F ; z = Γ(c)F ; z = n n zn c c (c) n! n=0 n converges only for z < 1, but it can be regarded as a large-c asymptotic series in much larger complex z-domainsj (cf.j [4, 15.12(ii)]). Thus, the representation (1.7) is very convenient since it is possible to obtain a large-νxasymptotic expansion by replacing the hypergeometric function with its hypergeometric series. This asymptotic expansion will be valid in the sector arg ν π δ j j ≤ − (< π) and for ξ 1, sinh ξ " (> 0). Error bounds for this expansion will then follow from the more general2 results D j we shallj ≥ prove for the hypergeometric series (see Section2). Before stating our main results, we introduce some notation. We define, for any n 0, ≥ 1 1 (4µ2 12)(4µ2 32) (4µ2 (2n 1)2) µ + µ a (µ) = − − ··· − − = 2 − n 2 n ; n 8nn! ( 2)n n! − and let ( p sin(πµ) if ξ > 0; pπΓ 2 + 1 (1.9) C(ξ; µ) = πiµ χ(p) = p 1 ; p > 0: ie∓ if 0 < ξ < π; Γ + ± ±= 2 2 The main large parameter asymptotic expansions we prove in this paper are as follows. 4 G. NEMES AND A. B. OLDE DAALHUIS Legendre functions for large ν and fixed µ. Assume that ξ , sinh ξ " (> 0) and 2 D1 j j ≥ µ C is bounded. Then the associated Legendre functions have the inverse factorial expansions 2 ν+ 1 ξ 1 n ( 2 ) 1 ξ µ e X Γ ν n + 2 e− P (cosh ξ) an(µ) − − ν ∼ p2π sinh ξ Γ(ν µ + 1) sinh ξ (1.10) n=0 − (ν+ 1 )ξ 1 ξ n C(ξ; µ)e− 2 X1 Γ ν n + e + a (µ) − 2 ; p2π sinh ξ n Γ(ν µ + 1) sinh ξ n=0 − 1 ξ n r 1 1 πiµ µ π (ν+ )ξ X Γ ν n + 2 e (1.11) e− Q (cosh ξ) e− 2 a (µ) − ; ν ∼ 2 sinh ξ n Γ(ν µ + 1) sinh ξ n=0 − as ν + , with ν being bounded.
Details
-
File Typepdf
-
Upload Time-
-
Content LanguagesEnglish
-
Upload UserAnonymous/Not logged-in
-
File Pages32 Page
-
File Size-