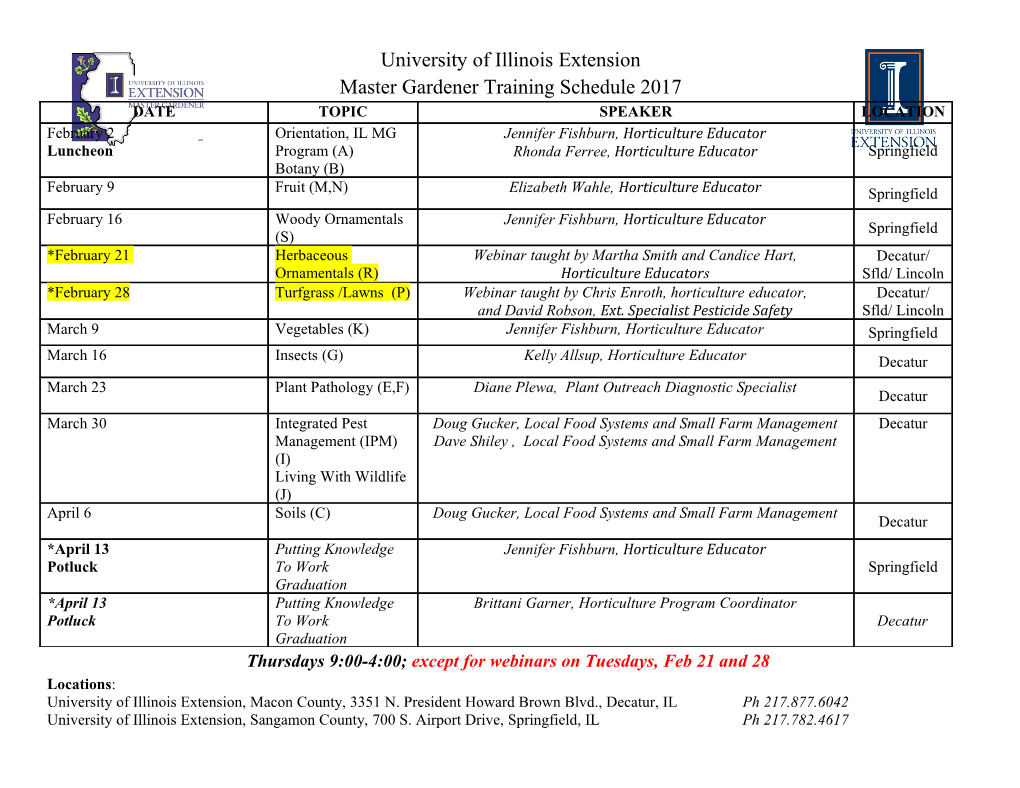
ATOM INTERFEROMETRY IN A 10 M FOUNTAIN A DISSERTATION SUBMITTED TO THE DEPARTMENT OF PHYSICS AND THE COMMITTEE ON GRADUATE STUDIES OF STANFORD UNIVERSITY IN PARTIAL FULFILLMENT OF THE REQUIREMENTS FOR THE DEGREE OF DOCTOR OF PHILOSOPHY Alex Sugarbaker August 2014 © 2014 by Alexander Franz Sugarbaker. All Rights Reserved. Re-distributed by Stanford University under license with the author. This work is licensed under a Creative Commons Attribution- Noncommercial 3.0 United States License. http://creativecommons.org/licenses/by-nc/3.0/us/ This dissertation is online at: http://purl.stanford.edu/kd753jv6128 ii I certify that I have read this dissertation and that, in my opinion, it is fully adequate in scope and quality as a dissertation for the degree of Doctor of Philosophy. Mark Kasevich, Primary Adviser I certify that I have read this dissertation and that, in my opinion, it is fully adequate in scope and quality as a dissertation for the degree of Doctor of Philosophy. Peter Graham I certify that I have read this dissertation and that, in my opinion, it is fully adequate in scope and quality as a dissertation for the degree of Doctor of Philosophy. Giorgio Gratta Approved for the Stanford University Committee on Graduate Studies. Patricia J. Gumport, Vice Provost for Graduate Education This signature page was generated electronically upon submission of this dissertation in electronic format. An original signed hard copy of the signature page is on file in University Archives. iii Abstract This thesis presents experimental results from the Stanford 10 m atom drop tower. We use atomic physics and laser spectroscopic techniques to test both general relativity and quantum mechanics. By dropping different types of atoms and observing their free-fall accelerations, it will be possible to test the equivalence principle and other general relativistic effects in the lab. By observing coherence after splitting an atom by up to 8.2 cm, we have probed the quantum-to-classical transition with increasingly macroscopic superposition states. I will discuss our development of long-baseline atom interferometry with 87Rb. We have demonstrated a record interrogation time of 2 T = 2:3 s. With 2~k atom optics, we infer an acceleration sensitivity of 6:7 10−12g per shot. We have also measured × Earth's rotation rate with 200 nrad/s precision and the direction of true North with 10 mdeg precision. I will show how spatially resolved detection and atomic point sources enable multiaxis inertial sensing. This work has required advanced de Broglie wave atom optics. We have devel- oped magnetic and optical-dipole lensing techniques capable of cooling atoms in two +50 dimensions to an effective temperature of less than 50−30 pK. We have also imple- mented large momentum transfer beamsplitters and mirrors (up to 12~k) for increased sensitivity and wavepacket separation. Many of the techniques presented here are broadly relevant to atom-interferometric precision measurement, from compact sensors of gravity, rotation, and acceleration to space-based gravitational wave detectors. iv Acknowledgments First and foremost, I thank my advisor Mark Kasevich. His optimism has pushed us to achieve the results presented in this thesis. His intuition and experience enable him to quickly give us many options to solve problems and explore new directions. He is a founder and visionary leader in our field, as evidenced by his inspiring keynote lecture at the 2013 International School of Physics \Enrico Fermi" on Atom Interferometry. I also thank my examination committee (Mark Kasevich, Peter Graham, Giorgio Gratta, Leo Hollberg, and Philip Levis) for their time. All of the results presented in this thesis have been a collaborative effort of the EP Team, which has been a big group for an atomic physics experiment because of the 10 m scale and sheer awesomeness of the apparatus. Figure1 shows most of the experimental and theoretical professors, postdocs, and PhD students that have contributed to the project. I've learned a lot from and with all of them during my time in graduate school. I would also like to acknowledge valuable discussions with our NASA collaborators, esp. Babak Saif. The rest of the KTeam has been a great group, whether on the ski slopes, at the mandatory Patxi's farewell dinners, during the bizarrely elected movie nights, or anytime I need to borrow the RF power meter. They are: Tim, Thomas, Philippe, Onur, Seth, David, Josh, Patrick, John, Brannon, Susannah, The Brothers Hammer, Nils, Mike, Sean, Sheng-wey, Christine, Kaspar, Catherine, Chris, Jan, Xinan, Jongmin, Hui-Chun, Chetan, Geert, Jason, Mark, Raj, Dave, Igor, Nick, Olaf, Christoph, Gunnar,1 and other visitors, undergrads, and high school students. Working in the Varian Physics Building wouldn't have been possible without help 1 1 12 1 3 Here's a puzzle for sneaky Belgians: 12842 43 421443y 138b9 2 3 43 4 53144 24 11 441. 2.13. v Figure 1: From top/left: Alex, Tim, Jason, Susannah, Sheng-wey, Mini-Me, Tiny Tim, Lego Jason, Short(er) Susannah, Harry Potter, Mark, Lego Mark, Philippe, L´ego Philippe, Jan, Leg¨oJan, Dave, Gunnar, a lumberjack, Lego'nar (aka \the Dread Pirate Brickberts," aka \the real stud of Lego"), Surjeet, Lego Surjeet, Chris, Christine, Christerbricks, Asimina, Lego Asimina, Mark, Savas, Jason, Peter, Lego Mixup (a little-known late-90s pop band). Thanks to David Pickett for designing and assembling the Lego caricatures. vi from its wonderful administrative staff: Ping, Sha, Beth, Liz, Lucy, Stewart, and Khoi. I've been fortunate in that our lab is next to the machine shop: Karlheinz, John, Mehmet, Matt, and Scott have all helped us build the 10 m interferometer. Teaching has been a big part of grad school, and I thank Chaya, Rick, and Elva for the opportunities and support to explore new teaching methods and convey the excitement of physics to Stanford undergrads. I'm also grateful to Maria for marshaling us all through grad school { I'm sure she's glad the year of 46 is finally out the door. I would also like to acknowledge financial support throughout graduate school from an Anne T. and Robert M. Bass Stanford Graduate Fellowship (SGF), from the National Science Foundation Graduate Research Fellowship Program (NSF GRFP), and from a National Defense Science and Engineering Graduate (NDSEG) Fellowship from the U.S. Department of Defense Air Force Research Laboratory/Air Force Office of Scientific Research (AFRL/AFOSR). Thanks to my friends throughout the years for their support and distractions (Wellingtonians, Shoreyites, Colonizers, SPSers, Science Buses, PhysGrads, Rains Plants, Space Odysseyers, Palmertons, and other Roommatesn). Finally, I thank my parents, grandparents, and other family for their years of support. I didn't realize until partway through graduate school how many similarities there are between my research and that of both of my parents, as evidenced by these (suggestively abbreviated) quotes from some of their papers: The relativistic heavy ion collider was built to create and investigate . matter at energy densities unprecedented in a laboratory setting { matter so hot that neutrons, protons and other hadrons are expected to \melt". [These] extreme conditions . afford RHIC the exciting scientific opportunity to discover the properties of matter under conditions believed to pertain during a critical, though fleeting, stage of the universe's earliest development following the big bang. The properties of such matter test fundamental predictions of quantum chromodynamics . [1] Standard T2∗ measurements (e.g., using conventional multiecho gradient-echo (GE) sequences) are affected by macroscopic static magnetic field (B0) inhomogeneities . The multi-GE slice excitation profile imaging (mGESEPI) method was developed for T2∗ measurements in the presence of macroscopic B0 inhomogeneity, but it requires excessive acquisition times . In this paper a more efficient technique . is proposed. [2] vii Contents Abstract iv Acknowledgmentsv 1 Introduction1 1.1 Atom Interferometry...........................2 1.1.1 Raman Mach-Zehnder Interferometer..............2 1.1.2 Interferometer Phase Shifts...................5 1.2 Precision Gravity Measurements.....................9 1.2.1 Test of the Equivalence Principle................ 10 1.2.2 Other Laboratory Tests of General Relativity......... 12 1.2.3 Gravitational Waves....................... 13 1.3 Macroscopic Quantum Mechanics.................... 16 1.4 Organization of this Thesis........................ 19 2 Experimental Apparatus and Methods 21 2.1 Experimental Overview.......................... 21 2.2 Interferometer Region (Free Fall).................... 25 2.2.1 Vacuum System.......................... 26 2.2.2 Magnetic Shielding........................ 30 2.2.3 Atom Optics Lasers........................ 33 2.2.4 Primary Telescope........................ 41 2.2.5 Rotation Compensation System................. 42 2.3 Atom Source (Cooling).......................... 45 viii 2.3.1 Magneto-Optical Trap...................... 45 2.3.2 Magnetic Trap Evaporation................... 48 2.3.3 Optically Plugged Quadrupole Trap............... 52 2.3.4 TOP Trap............................. 53 2.3.5 Magnetic Lens........................... 57 2.3.6 State Preparation......................... 57 2.4 Launch................................... 58 2.4.1 Coherent Optical Lattice Launch................ 58 2.4.2 Lattice Beam Geometry..................... 60 2.4.3 Optimizing Lattice Launch Parameters............. 62 2.5 Detection................................
Details
-
File Typepdf
-
Upload Time-
-
Content LanguagesEnglish
-
Upload UserAnonymous/Not logged-in
-
File Pages162 Page
-
File Size-