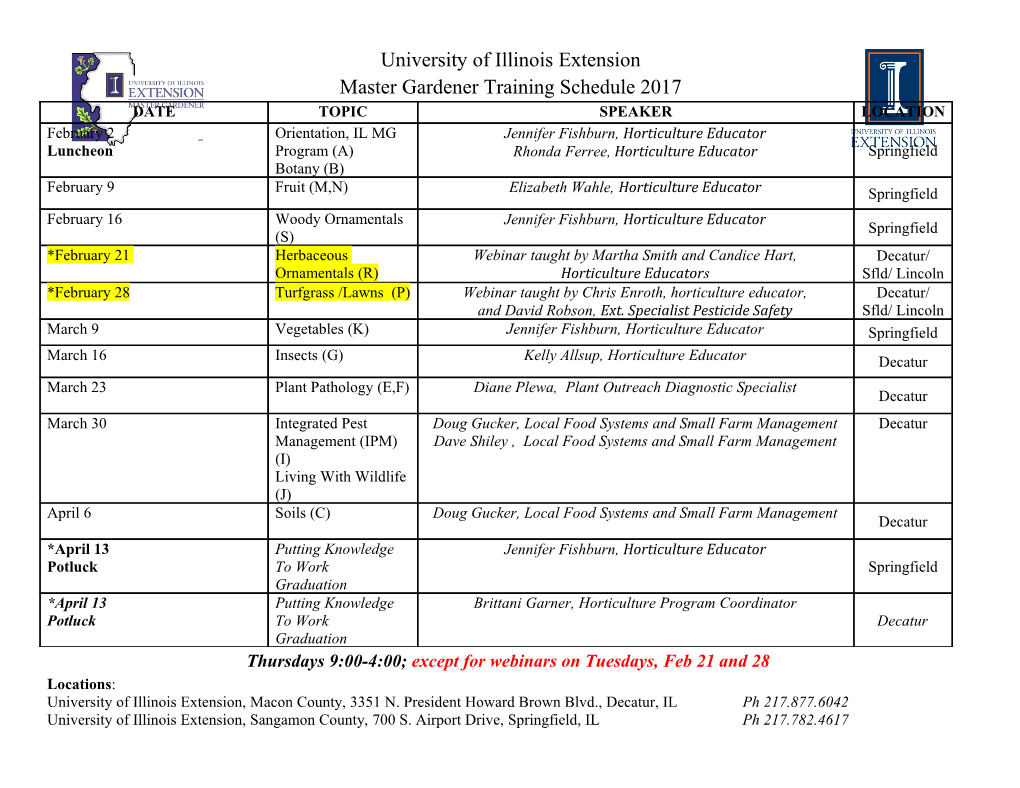
“[…] patience is sometimes the best policy” — Leonard, J.; Lygo, B.; Procter, G. Advanced Practical Organic Chemistry. Till Krypet List of Papers This thesis is based on the following papers, which are referred to in the text by their Roman numerals. I Efficient DNA Binding and Condensation Using Low Mole- cular Weight, Low Charge Density Cationic Polymer Am- phiphiles Mindemark, J.; Bowden, T. Macromolecular Rapid Communications 2010, 31, 1378–1382 II Low Charge Density Cationic Polymers for Gene Delivery: Exploring the Influence of Structural Elements on In Vitro Transfection Mindemark, J.; Tabata, Y.; Bowden, T. Accepted for publication in Macromolecular Bioscience III Synthesis and polymerization of alkyl halide-functional cyc- lic carbonates Mindemark, J.; Bowden, T. Polymer 2011, 52, 5716–5722 IV Diversity in cyclic carbonates: Synthesis of triazole- functional monomers using click chemistry Mindemark, J.; Bowden, T. Submitted manuscript Reprints were made with permission from the respective publishers. Contents 1 Introduction ......................................................................................... 11 1.1 Polymers ......................................................................................... 11 1.2 Polymers as biomaterials ................................................................ 12 1.2.1 Biodegradable polyesters and polycarbonates ....................... 13 1.2.2 Functional polymers .............................................................. 15 1.3 Cyclic carbonate monomers ............................................................ 16 1.3.1 Synthesis of 6-membered cyclic carbonates .......................... 16 1.3.2 Polymerization of 6-membered cyclic carbonates ................. 20 1.4 Click chemistry ............................................................................... 21 1.4.1 Cu(I)-catalyzed azide–alkyne cycloaddition .......................... 21 1.4.2 Click chemistry in monomers and polymers ......................... 22 1.5 Gene delivery .................................................................................. 23 1.5.1 Cationic polymers as delivery vectors ................................... 23 1.5.2 Structure–activity relationships ............................................. 25 2 Results and discussion ......................................................................... 26 2.1 Cationic polycarbonates for gene delivery ...................................... 26 2.1.1 Polymer synthesis .................................................................. 26 2.1.2 In vitro degradation ................................................................ 29 2.1.3 DNA binding and condensation ............................................. 31 2.1.4 In vitro gene delivery ............................................................. 36 2.2 Functional cyclic carbonate monomers ........................................... 40 2.2.1 Alkyl halide-functional cyclic carbonates ............................. 40 2.2.1.1 Monomer synthesis ..................................................... 40 2.2.1.2 Polymerization ............................................................ 42 2.2.1.3 Thermal properties and crystallinity ........................... 45 2.2.2 Triazole-functional cyclic carbonates .................................... 48 3 Concluding remarks and future perspectives ....................................... 53 4 Acknowledgements.............................................................................. 58 5 Svensk sammanfattning ....................................................................... 61 6 References ........................................................................................... 64 Abbreviations CDCl3 Deuterated chloroform CDI 1,1’-Carbonyldiimidazole CuAAC Cu(I)-catalyzed azide–alkyne cycloaddition DCM Dichloromethane DiPEA Diisopropylethylamine DMAE 2-(Dimethylamino)ethanol DMAEB 2-(Dimethylamino)ethyl benzoate DMF N,N-Dimethylformamide DP Degree of polymerization DSC Differential scanning calorimetry FT-IR Fourier transform infrared spectroscopy GPC Gel permeation chromatography Mn Number-average molecular weight Mw Weight-average molecular weight NMR Nuclear magnetic resonance PBS Phosphate-buffered saline PDI Polydispersity index PEG Poly(ethylene glycol) PEI Polyethylenimine PLLA Poly(L-lactic acid), poly(L-lactide) PTMC Poly(trimethylene carbonate) ROP Ring-opening polymerization Tc Crystallization temperature Td Degradation temperature Tg Glass transition temperature Tm Melting temperature TGA Thermogravimetric analysis THF Tetrahydrofuran TMC Trimethylene carbonate Scope of the thesis Functional polymers are essential for several applications of macromolecular materials. From a polymer chemistry point of view, the synthesis of func- tional polymer structures is limited by a combination of polymerization me- thods, monomers and post-polymerization functionalization strategies. The work presented in this thesis has been focused on the last two points for the synthesis of functional polycarbonates, a class of degradable polyesters widely used in biomaterials applications. In Paper I and Paper II, end-group functionalization was used to create cationically functional poly(trimethylene carbonate) structures capable of interacting with DNA. These structures, that are radically different from typical gene delivery materials, were studied in terms of biodegradability and the formation of polymer/DNA complexes. After confirming DNA bind- ing and condensation, the in vitro transfection efficienciy, cytotoxicity and physicochemical properties of the complexes were further characterized. The results obtained were used to deduce structure–activity relationships that are key to the design and development of efficient vectors for non-viral gene delivery. In Paper III, alkyl halide-functional homo- and copolycarbonates were synthesized through polymerization of alkyl halide-functional cyclic carbo- nate monomers and the properties of the polymers were characterized with a particular focus on semicrystallinity. The functional monomers were used in Paper IV as a starting point for the further synthesis of triazole-functional monomers and polymers using click chemistry. Altogether, this thesis presents a selection of strategies for the synthesis of functional polycarbonates. The insights gained from the presented work will act to expand our knowledge on factors that are essential to the perfor- mance of non-viral vectors for gene delivery as well as broaden the diversity of functional cyclic carbonate monomers for ring-opening polymerization. 1 Introduction 1.1 Polymers A polymer is, according to the IUPAC definition, ‘a substance composed of macromolecules’. Macromolecules are, as the name implies, large molecules with a high molecular weight that are characterized by being composed of repeating units of a low molecular weight. The repeating units that constitute a macromolecule typically derive from those monomers that, in the process of polymerization, combine to form the macromolecular structure. The origin of the term ‘polymer’ can be traced to the 19th century and the Swedish chemist Jöns Jakob Berzelius, although at that time it was used in a somewhat different context, referring not to macromolecules but instead to a form of isomerism.1 According to the Berzelius definition, polymerism is the concept of two compounds having the same relative chemical composition, e.g. CH, but different structures and thus different chemical and physical properties, e.g. acetylene (C2H2) and benzene (C6H6). While this definition would include many modern polymers with compositional formulas that are multiples of those of the corresponding monomers, such as polyacetylene ([C2H2]n) being a polymer of acetylene, the original definition did not con- cern macromolecules, since at that time it was believed that such structures simply could not exist. Many properties that are typical for polymers, such as high viscosities, slow rates of diffusion and a refusal to crystallize, were indeed observed, but these were attributed to the presence of large particles that were assembled by physical rather than chemical bonds.2 It was not until the 1920s that, thanks to the efforts of German chemist Hermann Staudinger, the scientific community started to realize that sub- stances that appeared to consist of very large molecules in fact did consist of very large molecules, referred to as macromolecules.3 Although there clearly is a conceptual difference between polymers and macromolecules, today these terms are often used interchangeably. Contributing to the relatively late acceptance of macromolecules is the fact that polymers, in contrast to low-molecular-weight compounds, are not necessarily well-defined in terms of structure and molecular weight. Instead, a polymer is typically a mixture of individual macromolecular chains that have different numbers of repeating units and, hence, different molecular weights. This leads to materials with varying properties depending on the exact composition. 11 The properties of polymers may be controlled through a combination of monomer composition, polymerization methods and post-polymerization treatment. This gives polymer chemistry a flexibility and versatility that enables the synthesis of a wide range of materials with different properties. Today, polymers such as polyethylene, polypropylene and polystyrene are present in a range of everyday items. Through the careful use of polymer chemistry it is possible to design and
Details
-
File Typepdf
-
Upload Time-
-
Content LanguagesEnglish
-
Upload UserAnonymous/Not logged-in
-
File Pages74 Page
-
File Size-