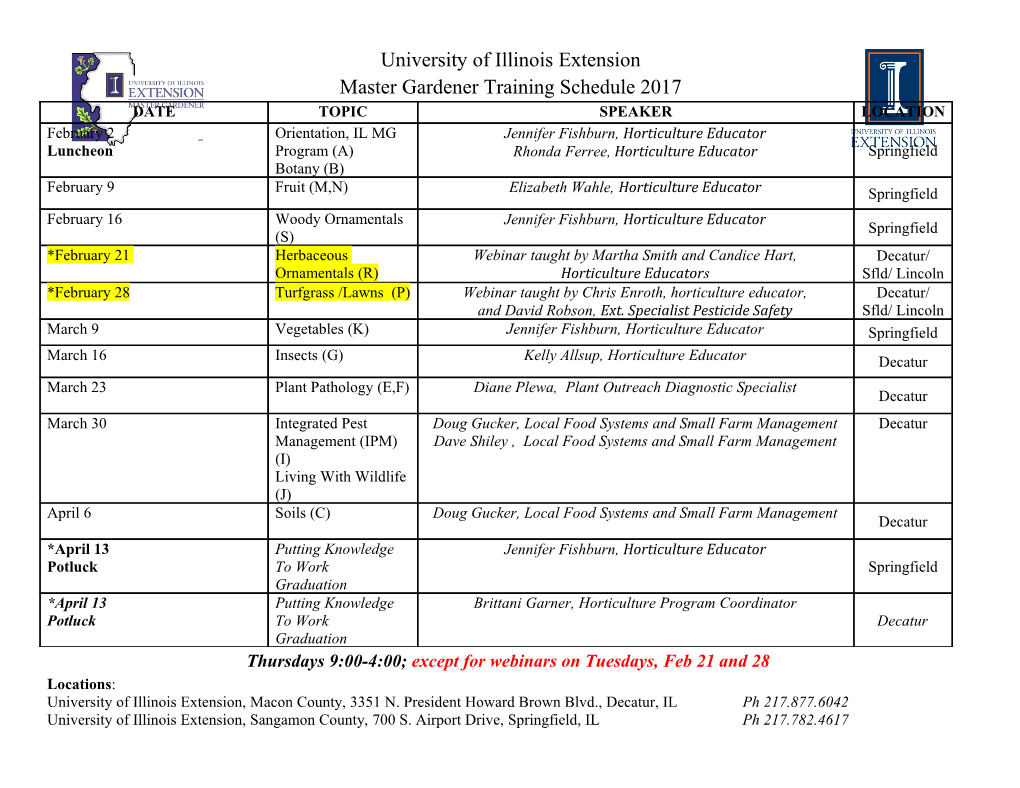
RESEARCH GLOBAL CARBON CYCLE We started our soil warming study in 1991 in an even-aged mixed hardwood forest stand at the Harvard Forest in central Massachusetts (42.54°N, 72.18°W), where the dominant tree Long-term pattern and magnitude of species are red maple (Acer rubrum L.) and black oak (Quercus velutina Lam.). The soil is a soil carbon feedback to the climate stony loam with a distinct organic matter–rich forest floor. (See the supplementary materials system in a warming world for more information on the site’s soils, climate, and land-use history.) The field manipulation contains 18 plots, each J. M. Melillo,1* S.D. Frey,2 K. M. DeAngelis,3 W. J. Werner,1 M. J. Bernard,1 6 × 6 m, that are grouped into six blocks. The F. P. Bowles,4 G. Pold,5 M. A. Knorr,2 A. S. Grandy2 three plots within each block are randomly as- signed to one of three treatments: (i) heated plots In a 26-year soil warming experiment in a mid-latitude hardwood forest, we documented in which the average soil temperature is con- changes in soil carbon cycling to investigate the potential consequences for the climate tinuously elevated 5°C above ambient by the use system. We found that soil warming results in a four-phase pattern of soil organic matter of buried heating cables; (ii) disturbance con- decay and carbon dioxide fluxes to the atmosphere, with phases of substantial soil carbon trol plots that are identical to the heated plots loss alternating with phases of no detectable loss. Several factors combine to affect the except that they receive no electrical power; and timing, magnitude, and thermal acclimation of soil carbon loss. These include depletion of (iii) undisturbed control plots that have been left microbially accessible carbon pools, reductions in microbial biomass, a shift in microbial in their natural state (no cables). The heating Downloaded from carbon use efficiency, and changes in microbial community composition. Our results support method works well under a variety of moisture projections of a long-term, self-reinforcing carbon feedback from mid-latitude forests to the and temperature conditions (13). Here, we com- climate system as the world warms. pared carbon dynamics measured in the heated plots to those measured in the disturbance control large and poorly understood component sink to a carbon source is critically dependent on plots, so as to isolate heating effects from the of global warming is the terrestrial carbon the long-term temperature sensitivity of soil organic effects of cable installation (e.g., root cutting and http://science.sciencemag.org/ cycle feedback to the climate system (1). matter (SOM) decay (5–7) and complex carbon- soil compaction) (14). A Simulation experiments with fully coupled, nitrogen interactions that will likely occur in a We used a static chamber technique (14)to three-dimensional carbon-climate models warmer world (8–12). However, without long-term measure soil CO2 emission rates in the study suggest that carbon cycle feedbacks could sub- field-based experiments, the sign of the feedback 1 stantially accelerate or slow climate change over cannot be determined, the complex mechanisms Ecosystems Center, Marine Biological Laboratory, Woods Hole, MA 02543, USA. 2Department of Natural Resources the 21st century (2–4). Both the sign and magni- regulating that feedback cannot be quantified, and and the Environment, University of New Hampshire, Durham, tude of these feedbacks in the real Earth system models that incorporate the soil’sroleincarbon NH 03824, USA. 3Department of Microbiology, University of are still highly uncertain because of gaps in basic feedbacks to the climate system cannot be tested. Massachusetts, Amherst, MA 01003, USA. 4Research 5 understanding of terrestrial ecosystem processes. Here, we present results from a long-term (26-year) Designs, Lyme, NH 03768, USA. Graduate Program in Organismic and Evolutionary Biology, University of For example, the potential switch of the terres- soil-warming experiment designed to explore Massachusetts, Amherst, MA 01003, USA. trial biosphere from its current role as a carbon these feedback issues in an ecosystem context. *Corresponding author. Email: [email protected] on February 14, 2018 Fig. 1. Effect of soil warming on soil respiration Phase I Phase II Phase III Phase IV 1200 over 26 years. (A) Annual soil CO2 emissions ) from the control plots (black bars) and heated −1 1000 plots (gray bars). Asterisks denote years when the heated and control plots are significantly year 800 −2 Flux t 2 different (paired-sample tests or Wilcoxon 600 signed-rank tests as appropriate, n = (6, 6), −C m −C *P < 0.05; see supplementary materials). Hatched 2 400 Soil CO bars denote years when the heating system was 200 g CO inactive for the majority of the growing season. ( Error bars denote SEM (n = 6). (B) Four-year 0 rolling mean increase in soil CO2 emissions in the heated plots relative to the control plots, 1991 1992 1993 1994 1995 1996 1997 1998 1999 2000 2001 2002 2003 2004 2005 2006 2007 2008 2009 2010 2011 2012 2013 2014 2015 2016 excluding years when the heating system was **** ***** * ** *** * * inactive for the majority of the growing season. Flux 200 2 ) Error bars denote SEM derived from propagating 1 − SE estimates from (A) through the operations 150 year necessary to produce (B). See fig. S4 for annual Soil CO ) −2 100 changes in soil CO2 emissions in the heated plots relative to the control plots. ntrol −C m −C 50 2 d−Co CO 0 e g ( Heat −50 ( Δ 06−09 07−09 11−14 12−15 92−94 96−99 97−00 98−01 00−03 01−04 02−04 11−13 91−94 96−98 99−02 06−08 13−16* 08−09, 11 94, 96−97 04, 06−07 93−94, 96 09, 11−12 03−04, 06 Melillo et al., Science 358, 101–105 (2017) 6 October 2017 1of4 RESEARCH | REPORT plots monthly between April and November each year for 26 years (see supplementary materials). An ephemeral but significant soil respiration response to warming occurred over the experiment’s first decade (Fig. 1), with soil respiration greater in the heated plots than in the controls (phase I). In the next phase of the response, phase II (years 11 to 17), soil res- piration rates in the heated plots were gen- erally equal to or less than those in the control plots. During years 18 to 23 of the study (phase III), we observed a second trend reversal, with soil respiration once again higher in the heated plots. In the three most recent years (phase IV) of the study, 2014 to 2016, soil respiration rates in the heated plots were again equal to or less than those in the control plots. We partitioned soil respiration into its two components, root and microbial respiration. On the basis of field measurements of root respira- tion made between June and November 2009, Fig. 2. Four-year rolling mean cumulative modeled soil carbon losses from the full soil profile Downloaded from we developed a temperature-driven root respi- over 26 years of soil warming in the heated plots relative to the control plots. Relative soil ration model that we used to estimate root res- carbon losses are calculated as the difference in heterotrophic soil respiration between the heated n piration over the course of the experiment (see plots and the control plots. Error bars denote SEM, calculated for the heated ( = 6) and control n supplementary materials). We calculated microbial ( = 6) plots for each year and propagated through the necessary operations to produce this figure. respiration by difference, with microbial respira- tion equaling soil respiration minus root res- http://science.sciencemag.org/ piration (10). Our estimate is that two-thirds of the cumulative CO -C emitted from the plots over 2 Phase I (1991−2000) Phase II (2001−2007) the 26-year study has been microbial. By this analysis, we calculate a warming-induced soil ) carbon loss from the full soil profile over the 1 − 300 400 26-year study of 1510 ± 160 g C m−2,whichis hr equivalent to a 17% loss of the soil carbon found −2 in the top 60 cm of the soil at the start of the 200 Flux 2 experiment. With respect to timing, we estimate m −C 2 that about three-quarters of this soil carbon loss CO occurred during phase I, and the remaining quar- on February 14, 2018 mg CO ter during phase III. No measurable carbon ( +5°C +5°C loss occurred during either phase II or phase 0 100 200 300 400 0 100 IV (Fig. 2). 0 5 10 15 20 25 0 5 10 15 20 25 In both the control and heated plots, we made direct measurements of carbon stocks in the upper horizons of the soil profile—the distinct, organic Phase III (2008−2013) Phase IV (2014−2016) matter–rich surface horizon or forest floor and the top 30 cm of the mineral soil just beneath the ) −1 300 400 300 400 forest floor (fig. S1). We measured a carbon loss r h from the forest floor in response to soil warming 2 − −2 of 800 ± 300 g C m , which represents a 31% m 200 C Flux reduction in forest floor carbon stock over the 2 − 26-year study. With our direct measurements, we 2 CO O did not detect any statistically significant changes C 100 200 in the carbon stocks across the top 30 cm of the g m mineral soil horizon. However, combining our ( +5°C +5°C estimate of carbon loss from the full profile based 0 0 100 on the respiration measurements with the carbon 0 5 10 15 20 25 0 5 10 15 20 25 loss measured from the forest floor, we estimate Temperature (°C) Temperature (°C) that the warming-induced carbon loss from the mineralsoilinthefullsoilprofileoverthestudy Fig.
Details
-
File Typepdf
-
Upload Time-
-
Content LanguagesEnglish
-
Upload UserAnonymous/Not logged-in
-
File Pages5 Page
-
File Size-