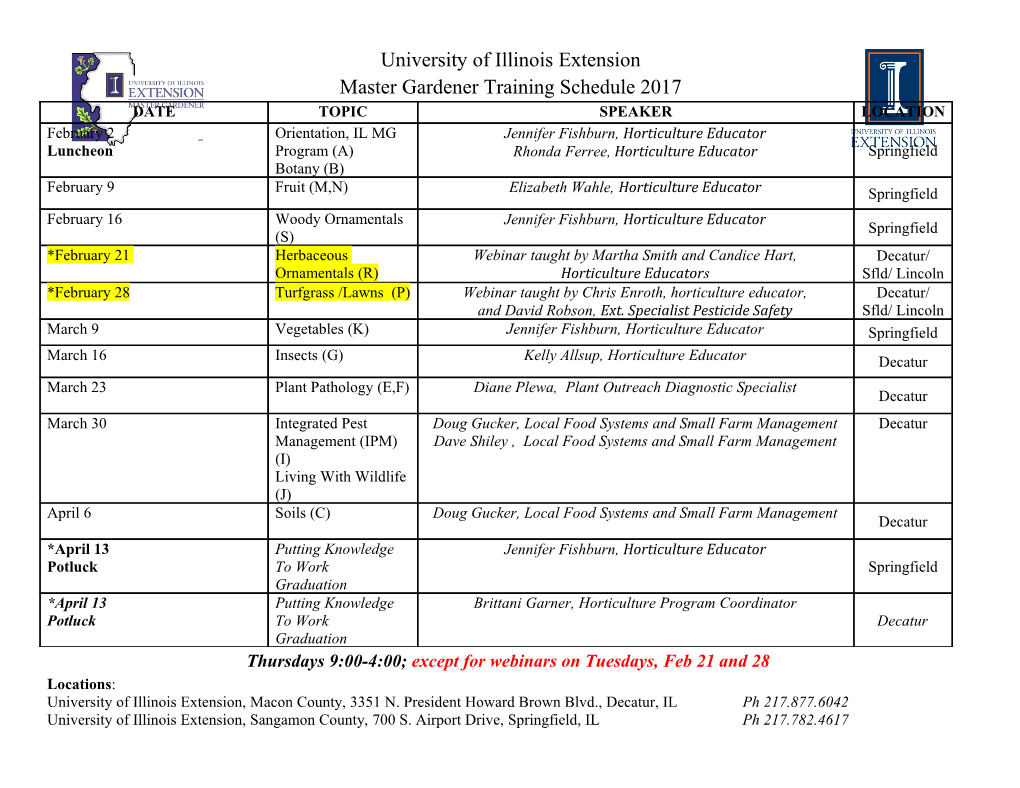
PUBLICATIONS AlllDM imOSQ NBS!R 84-2942 An Evaluation of Ettringite and Related Compounds for Use in Solar Energy Storage L. J. Struble and ?. W. Brown U.S. DEPARTMENT OF COMMERCE National Bureau of Standards National Engineering Laboratory Center for Building Technology Gaithersburg, MD 20899 July 1984 Progress Report issued October 1 9S4 — QC 100 .U56 84-2942 1984 Sponsored by: U.S. Department of Energy Office of Solar Heat Technologies Passive and Hybrid Solar Energy Division Washington, DC 20585 JfATIONAl BUHEAtf OF STANDABDS LIBRARY NBSIR 84-2942 AN EVALUATION OF ETTRINGITE AND RELATED COMPOUNDS FOR USE IN SOLAR ENERGY STORAGE L J. Struble and P. W. Brown U.S. DEPARTMENT OF COMMERCE National Bureau of Standards National Engineering Laboratory Center for Building Technology Gaithersburg, MD 20899 July 1984 Progress Report Issued October 1984 Sponsored by U.S. Department of Energy Office of Solar Heat Technologies Passive and Hybrid Solar Energy Division Washington, DC 20585 U.S. DEPARTMENT OF COMMERCE, Malcolm Baldrige, Secretary NATIONAL BUREAU OF STANDARDS. Ernest Ambler. Director AN EVALUATION OF ETTR INCITE AND RELATED COMPOUNDS FOR USE IN SOLAR ENERGY STORAGE Leslie J. Struble and Paul W. Brown Center for Building Technology National Bureau of Standards July 1, 1984 SUMMARY This report describes an investigation of ettringite and related phases for potential application in solar energy storage. The specific objective is to evaluate the potential of ettringite dehydration and rehydration as a phase change process for energy storage. Synthesis procedures have been developed, and a number of ettringite-type phases have been prepared. The heat capacity of each phase was approximately 0.3 calories per gram per degree Celsius. Studies of the dehydration of these phases at atmospheric pressure indicate that the material has good potential as a phase change material for solar energy storage. Dehydration occurred at temperatures in the range between approximately 30°C and 55°C, with changes in enthalpy ranging between 100 and 240 calories per gram of sample. In addition, ettringite was found to have a reversible hydrothermal reaction at approximately 50®C, with an enthalpy change of approximately 4 calories per gram sample. Future work during the remainder of this program will involve completing the work described in the present progress report. 1 I. INTRODUCTION Effective utilization of solar energy in the passive mode requires the utilization of materials in which either sensible or latent heat may be stored. One class of materials that holds promise for this application is hydrated salts, an example of which is ettringite, also called Candlot's salt, a hydrated calcium alumi no-sulfate that forms during the hydration of Portland cement. Therefore, a laboratory study is being carried out in order to evalu- ate ettringite and related phases for possible application in passive solar energy systems. Preliminary studies indicated that ettringite dehydration has potential for thermal energy storage (1). Further work, described in the present report, has focussed on the synthesis of ettringite and several related phases, and the determination of their thermal properties. A. ENERGY STORAGE MATERIAL Solar energy storage materials have two basic functions: to store solar energy, and to release it at the appropriate time. To carry out these functions, storage systems may rely on the specific heat of a material, and may also rely on a phase change which occurs in the material. In general, the amount of energy that a material is capable of storing and releasing is significantly greater if a phase change is involved. On the other hand, the phase change may bring about other changes, such as physical degradation, that limit the usefulness of the material in this application. Specific heat, or more precisely thermal capacity, is a fundamental and measurable property (though it varies somewhat with temperature). Values of specific heat, i.e. the thermal capacity of the material relative to that of water, range considerably. The thermal capacity of water is 1 calorie per gram per degree Celsius, while thermal capacities of solids are somewhat lower, typically a few tenths calorie per gram per degree. There is also a change in heat, or enthalpy, associated with any phase change — including melting, changes in crystal structure, dehydration, and dissolution. The enthalpy change may be positive, such that heat is consumed (endothermic) or negative, such that heat is released (exothermic). If the phase change is reversible, then the enthalpy change is also reversible. Therefore a reversible phase change may be used for solar energy collection. The phase change utilized most extensively is melting, and an example is the melting of Glauber's salt, * Na 2S04 10 H 20 . The change in enthalpy due to a phase change is a measurable property of the material (e.g., 80 calories per gram for the melting of ice, and 60 calories per gram for the melting of Glauber's salt). However, other parameters associated with phase changes are not fuiidamental properties. The kinetics of the change, and therefore the precise temperature at which the change occurs at a reasonable rate, may vary considerably with the nature of the sample — the amount of material, its particle size, etc. The temperature of a phase change is particularly important in passive solar devices, where the operating temperature is limited. Therefore, the kinetics 2 and the temperature of a phase change, as well as the enthalpy, must be considered in the evaluation of any material for use in solar energy collectors. Finally, there are several other considerations when evaluating a material for this application. For instance, the physical and chemical durabilities are critical. The material must be reasonably low in cost and readily available. It must be easily and safely handled. While these factors were not the focus of the present study, they were considered throughout the study. B. ETTRINGITE Ettringite is a hydrated calcium alumino-sulfate, [Ca5Al2(0H)i2](S04)3*26H20, which typically forms from reaction of Ca3Al206 with gypsum (CaS04»2H20) and water. The dehydration, or partial dehydration, of ettringite and related phases is the particular focus of these studies. The material is known to dehydrate partially at relatively low temperatures, and the dehydration is expected to be reversible. Therefore, ettringite dehydration offers potential as a phase change process for solar energy. The crystal structure of ettringite was described by Moore and Taylor (2) in 1970, and the structures of ettringite and related phases were subsequently reviewed by Taylor (3). The phase is hexagonal in structure, and typically occurs as a prism or needle, elongated parallel to the c-axis. The basic structural unit is a column of hydrated calcium aluminate hydroxide (Fig. la). The columns, positively charged, are arranged parallel to the c-axis of the crystal, with four such columns per unit cell (Fig. lb). The negative (SO4) ions and the remaining water molecules are found within the channels formed by these columns (Fig. Ic). The structural formula may thus be written [Ca3Al (0H)6*12H20]4(S04)6*4H20. The ettringite structure is very open, and considerable chemical substitution may occur. Based on the crystal structure, however, certain restraints on the substitution are expected. For instance, the substitution for the sulfate ions would be limited by the need to balance the positive charge of the columns, and by the available space within the channels. Substitution within the columns, on the other hand, would be limited primarily by the 6-fold coordination of the aluminum and calcium within the columns. Several types of chemical substitution have been reported within the channel sites. For instance, CO3 may replace SO4, either partially (3) or completely (4). In an early reference, Flint and Wells (5) reported substitution of Si03 for SO4. Finally, substitution of Cl , a monovalent ion, for SO4 has been reported (6). However, it is not clear from structural considerations whether monovalent ions may substitute for SO4. The crystal structure analysis suggests that there are not sufficient sites within the channel for the number of monovalent ions that are required for charge balance. Several substitutions have been described within the columns. Either Sr or Pb may substitute for Ca (3). The following elements have been reported to sub- stitute for Al: Ge (3); Fe, Mn (3,7); Ti (7); Cr (7,9,10); and Si (3,10). The mineral thaumasite is a doubly substituted ettringite, in which CO3 is 3 , partially substitutinq within the channel, and Si is substituting for A1 requirino 6-fold coordination of Si. • The ettringite structure thus contains three distinct types of water molecules: 1) water within the channel, 4 molecules per structural unit, 2) water bound to the columns, 48 molecules per structural unit, and 3) water as hydroxide ions on the columns, 12 molecules per structural unit. It is reasonable to expect the conditions for dehydration and the structural changes associated with(11) dehydration to be different for each of the three types of water. Dehydration may be carried out at room temperature and atmospheric pressure by reducing the partial pressure of water in the system. As reviewed by Lea , this dehydration is reported to proceed with no change in X-ray diffrac- tion pattern until there are 36 molecules of water per structural unit. This loss presumably corresponds to the loss of the 4 molecules of water from within the channel and the loss of 24 of the 48 molecules that are bound as water on the columns. Further dehydration causes a change in the X-ray diffraction pattern, i.e. a shift in the basal peak from 0.97 nm to 0.73 nm, until 16 molecules of water remain, corresponding to loss of most of the remaining water from the channels.
Details
-
File Typepdf
-
Upload Time-
-
Content LanguagesEnglish
-
Upload UserAnonymous/Not logged-in
-
File Pages52 Page
-
File Size-