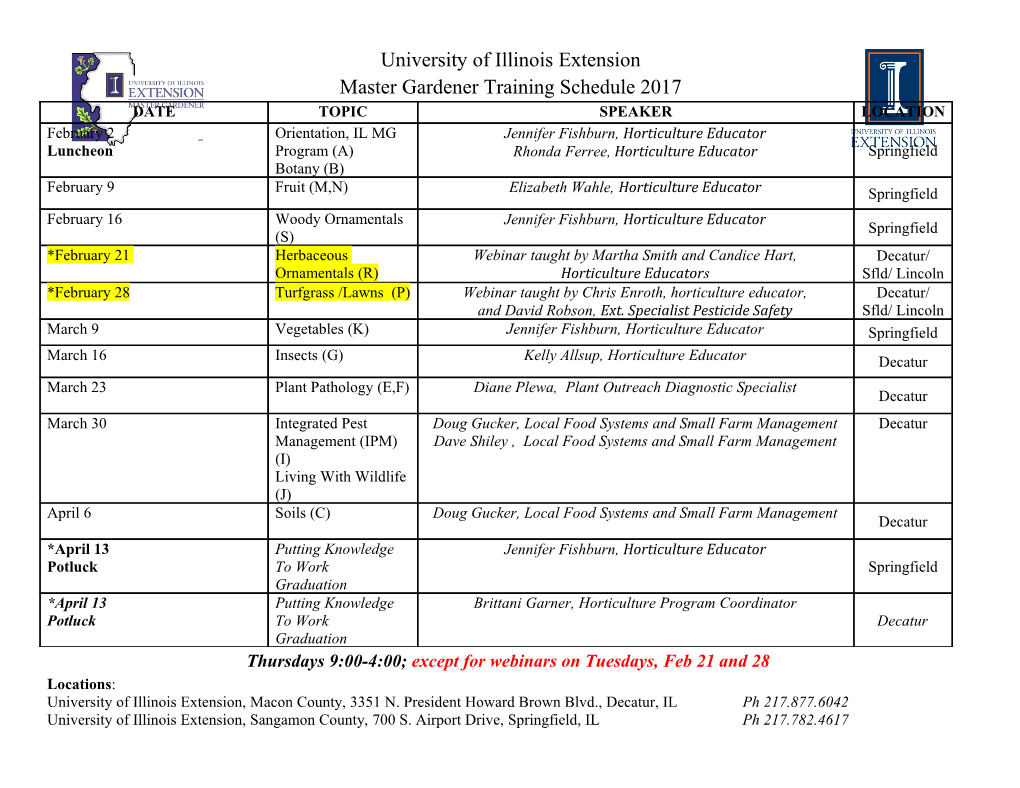
REVIEWS Building the Himalaya from tectonic to earthquake scales Luca Dal Zilio 1 ✉ , György Hetényi 2, Judith Hubbard3 and Laurent Bollinger4 Abstract | Convergence of the Indian Plate towards Eurasia has led to the building of the Himalaya, the highest mountain range on Earth. Active mountain building involves a complex interplay between permanent tectonic processes and transient seismic events, which remain poorly understood. In this Review, we examine the feedbacks between long-term tectonic deformation (over millions of years) and the seismic cycle (years to centuries) in the Himalaya. We discuss how surface morphology of the Himalaya indicates that the convergence is largely accommodated by slip on the Main Himalayan Thrust plate boundary fault, which developed in the roots of the mountain range over millions of years. At shorter (decadal) timescales, tectonic geodesy reveals that elastic strain is periodically released via earthquakes. We use examples from earthquake cycle models to suggest that partial ruptures could primarily occur in the downdip region of the Main Himalayan Thrust. Great (Mw 8+) Himalayan earthquakes are more commonly associated with complete megathrust ruptures, which release accumulated residual strain. By synthesizing numerous observations that co-vary along strike, we highlight that tectonic structures that developed over millions of years can influence stress accumulation, structural segmentation, earthquake rupture extent and location, and, consequently, the growth of the mountain range. orogen Orogen The Himalayan , standing at 7,000–8,000 m, rep- landscape modification and fluid migration). These A belt of the Earth’s crust resents a striking topographic boundary between the transient processes are impacted by structural geome- involved in the formation of near-sea-level Ganges and Brahmaputra plains to tries, lithologies, stress states and the presence of fluids, mountains, owing to tectonic the south and the 4,500–5,000-m-high Tibetan Plateau which often evolve over long timescales. plate convergence. to the north. The Himalaya are underlain by one of the Long-term deformation processes, therefore, influ- largest continental megathrusts on Earth, and, conse- ence all parts of the seismic cycle, including interseismic quently, the region experiences large earthquakes1 that stress-strain accumulation, seismic slip events (earth- cause extensive damage and death tolls in both the quakes), post-seismic deformation and non-linear Himalaya and the densely populated foreland plains (for viscous deformation at depth5. In turn, deformation example, 2015 Mw 7.8 Gorkha, 2005 Mw 7.6 Kashmir, across multiple earthquake cycles integrates to pro- 1Seismological Laboratory, 1950 Mw 8.5–8.7 Assam, 1934 Mw 8.4 Bihar–Nepal, duce long-term deformation and shapes tectonic struc- California Institute of 1905 Mw 7.8 Kangra, 1897 Mw 8.2–8.3 Assam–Shillong tures, from the topography to the roots of the orogen. Technology, Pasadena, California, CA, USA. Plateau). However, large Himalayan earthquakes have Furthermore, crustal deformation and topography are long recurrence intervals2 (hundreds of years), and the influenced by climate and surface denudation6–10, and, in 2Institute of Earth Sciences, University of Lausanne, limited instrumental record leaves large gaps in our sci- turn, topography and weathering can alter monsoonal Lausanne, Switzerland. entific understanding of how the long-term deformation patterns and long-term climate11,12. These multiple feed- 3Earth Observatory of links to and informs earthquake hazard. back cycles result in a non-linear, dynamically evolving Singapore, Nanyang Over geological timescales (104–107 years), crustal system that is coupled across a large number of spatial Technological University, deformation is usually described as a continuous, quasi- and temporal scales. Singapore, Singapore. steady process accommodated by a combination of In this Review, we discuss the interplay between the 4 Commissariat à l‘Energie viscous deformation at depth and brittle deformation long-term tectonics and short-term seismicity across Atomique et aux Energies 3 Alternatives (CEA), Arpajon, in the upper crust . However, the occurrence of strong the Himalayan range, and we describe how such interplay France. (6 < Mw < 7), major (7 < Mw < 8) and great (Mw 8+) affects the seismic behaviour and topography. As they are ✉e-mail: [email protected] earthquakes attests to the episodic nature of upper crus- subaerial, the Himalaya are one of the best places on Earth 4 https://doi.org/10.1038/ tal shortening and associated transient processes (such to observe and analyze the geologic evolution and natural s43017-021-00143-1 as dynamic weakening, viscous flow, post-seismic creep, hazards of a mountain belt. We discuss how large-scale NATURE REVIEWS | EARTH & ENVIRONMENT 0123456789();: REVIEWS 29 Key points called the South Tibetan Detachment . Rocks north of the South Tibetan Detachment are known as the • The Himalayan mountain belt is a unique subaerial orogenic wedge characterized Tethyan Himalaya, and consist of late Precambrian to by tectonically rapid, ongoing crustal shortening and thickening, intense surface early Palaeozoic sedimentary and metasedimentary denudation and recurrent great (Mw 8 ) earthquakes. + rocks29,30 and thick Permian to Cretaceous continental • The history of the orogen has been investigated from long (million-year) to short margin sequences16,31. The southern boundary of the (seconds to days) timescales using a variety of geological and geophysical techniques. High Himalaya is defined as a transition from steeper, • The magnitude 7.8 Gorkha earthquake and aftershocks were monitored by extensive higher topography in the north to a lower topography local geophysical networks, providing a unique set of observations of a major in the Lesser Himalaya to the south. The High Himalaya Himalayan earthquake and the Himalayan seismic cycle. exposes the Greater Himalayan crystalline sequence and, • Observations across the Himalaya reveal along-strike segmentation patterns at in some regions, parts of the largely Proterozoic meta- various temporal scales, controlled by inherited tectonic complexities developed over millions of years. sedimentary Lesser Himalayan sequence, which crops out from the Main Boundary Thrust (MBT) in the south to • Developing a complete understanding of deformation across timescales from 16,32 (Fig. 2a) seconds to millions of years requires an integrated, interdisciplinary effort. the Main Central Thrust (MCT) in the north . Geographically, the Lesser Himalaya domain is bounded by the MBT in the south to the MCT in the and long-term tectonic deformations contribute to struc- north and exposes Lesser Himalayan sequence rocks31. tural segmentation and earthquake generation, and how We note that Lesser Himalayan sequence rocks can be the Himalayan topography responds to stress pertur- found in both the Lesser and the High Himalaya, which bations across different stages of the seismic cycle. We are defined based on structure and topography, rather identify current knowledge gaps and future opportu- than lithology. To the south, the Sub-Himalaya is the nities towards better understanding how the Himalaya youngest part of the system, spanning from the frontal deform in space and time, which has implications for fault (the Main Frontal Thrust, or MFT) to the MBT; in seismic hazard. Although the features described in this this case, the stratigraphic and geographic definitions Review are based on observations from the Himalaya, the coincide. The Sub-Himalaya is built out of Siwaliks sed- interaction of multiscale processes is applicable to that in imentary foreland basin sediments and records the last other continent–continent collision zones. couple of million years of shortening history33. Himalayan shortening has been largely accommo- Long-term tectonic deformation dated along three main fault systems: the MFT, the MBT The Himalaya are a natural laboratory that provide a and the MCT (Fig. 2b). The most recently active faults are unique opportunity for studying mountain-building those in the Sub-Himalaya, which contains between 1 processes, earthquakes and deep dynamics related to and ~5 subparallel fault strands34. Together, these faults continent–continent collision. Understanding these are sometimes called the MFT system, with the MFT processes requires reconstruction of both crustal short- being the southernmost and youngest fault in the set. ening patterns (developed over millions of years) and The MFT is also the most active surface-breaching fault present-day crustal structures. In this section, we dis- associated with the Himalaya35. The MBT is roughly cuss the geological architecture of the Himalayan range parallel to the range front, began to slip at ~11 Ma and and review how geophysical explorations are used to was active during the Pleistocene36. The MCT is older: investigate the structure and tectonic processes at work. it formed at ~22–18 Ma and might have remained epi- sodically active37 since. As the MCT is refolded, its pri- Geological background. Over the last 70 to 50 million mary trace lies within the High Himalaya, but it is also years, northward drift of India and its collision with exposed around klippen within the Lesser Himalaya. Seismic cycle Eurasia has led to the construction of the Himalayan arc The MCT records the bulk of geological shortening Repeating process during and Tibetan Plateau13–18 (Fig. 1). The Tibetan Plateau, as in the
Details
-
File Typepdf
-
Upload Time-
-
Content LanguagesEnglish
-
Upload UserAnonymous/Not logged-in
-
File Pages18 Page
-
File Size-