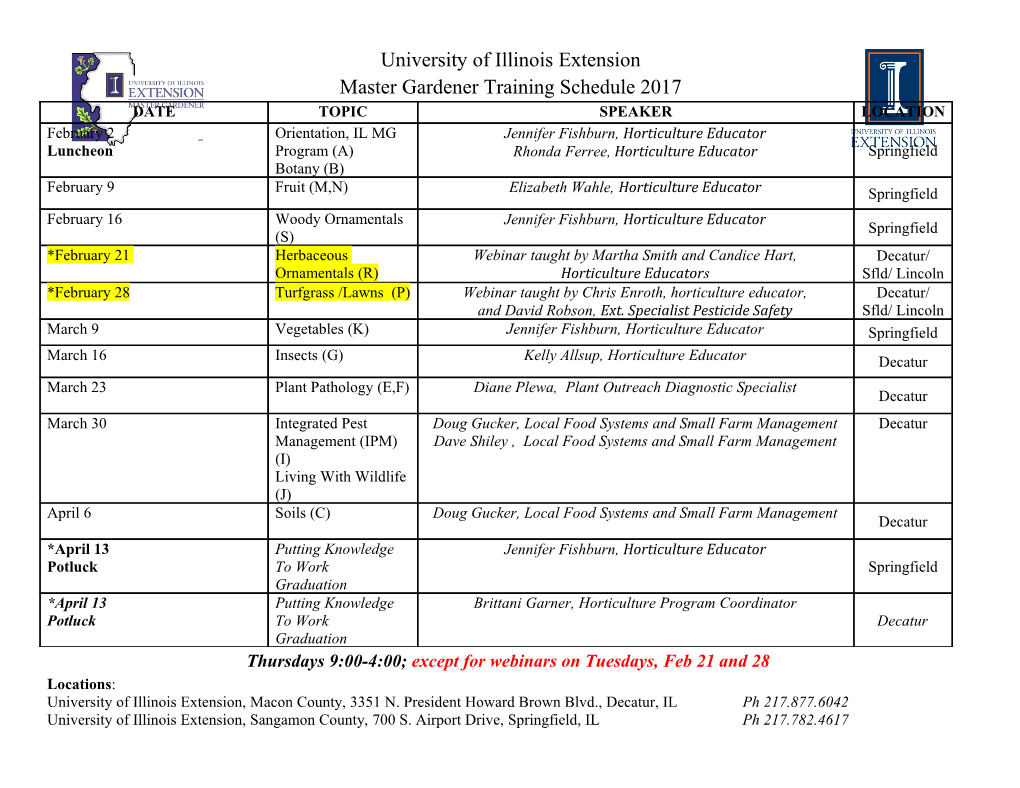
Bulletin of the Seismological Society of America, Vol. 102, No. 1, pp. 236–251, February 2012, doi: 10.1785/0120110041 A New Perspective on the Geometry of the San Andreas Fault in Southern California and Its Relationship to Lithospheric Structure by Gary S. Fuis, Daniel S. Scheirer, Victoria E. Langenheim, and Monica D. Kohler* Abstract The widely held perception that the San Andreas fault (SAF) is vertical or steeply dipping in most places in southern California may not be correct. From studies of potential-field data, active-source imaging, and seismicity, the dip of the SAF is significantly nonvertical in many locations. The direction of dip appears to change in a systematic way through the Transverse Ranges: moderately southwest (55°–75°) in the western bend of the SAF in the Transverse Ranges (Big Bend); vertical to steep in the Mojave Desert; and moderately northeast (37°–65°) in a region extending from San Bernardino to the Salton Sea, spanning the eastern bend of the SAF in the Trans- verse Ranges. The shape of the modeled SAF is crudely that of a propeller. If con- firmed by further studies, the geometry of the modeled SAF would have important implications for tectonics and strong ground motions from SAF earthquakes. The SAF can be traced or projected through the crust to the north side of a well documented high-velocity body (HVB) in the upper mantle beneath the Transverse Ranges. The north side of this HVB may be an extension of the plate boundary into the mantle, and the HVB would appear to be part of the Pacific plate. Introduction In the Southern California Earthquake Center Commu- as the scenario M 7.8 earthquake of ShakeOut (Perry nity Fault Model (CFM; Plesch et al., 2007), the dip of the et al., 2008). San Andreas fault (SAF) is 90° in all but one location, as there is little subsurface data to modify this default value. The one exception is the San Gorgonio Pass SGP area of the eastern Tectonic Setting Transverse Ranges (Fig. 1), where surface constraints and seismicity have been used to justify a nonvertical dip in the Through the Transverse Ranges, the SAF strikes obli- model. In this study, we constrain the dip of the SAF by ana- quely to relative plate motion with bends at either end of lyzing near-surface and subsurface data, including potential the oblique stretch (Fig. 1). The Los Angeles Region Seismic field, seismic-imaging, and seismicity observations, from a Experiment (LARSE) transects (Fig. 1, profiles 4 and 5), number of locations in southern California. Surprisingly, which were recorded across this oblique section of the the SAF is significantly nonvertical in most places, dipping SAF, reveal interpreted fluid-lubricated midcrustal decolle- away from the bends at either end of its stretch through the ments originating at the SAF and extending southward into Transverse Ranges, where it is oblique to plate motion. The the Pacific plate. Reverse faults that splay upward from these overall shape of the SAF is similar to that of a propeller. The decollements have given rise to destructive earthquakes in M fault appears to extend or project through the crust to join the the Los Angeles region, including the 1971 w 6.7 San M north side of the well known upper-mantle high-velocity Fernando and 1987 w 5.9 Whittier Narrows earthquakes body (HVB) beneath the Transverse Ranges that has been (Fuis et al., 2001, 2003). A crustal root is observed beneath imaged with teleseismic tomography and Rayleigh waves. the Transverse Ranges centered, or nearly centered, on the These findings, if confirmed in further studies, would have SAF (Kohler and Davis, 1997; Fuis et al., 2001; Godfrey important implications for understanding the tectonics and et al., 2002; Fuis et al., 2007). The tectonic interpretation of strong- ground-motion potential from SAF earthquakes, such this crustal structure is that the upper crust, above the decol- lements, responds to convergence in this oblique section of *Now at Department of Mechanical and Civil Engineering, California the SAF by vertical and lateral motion, whereas the lower Institute of Technology, Pasadena, California 91125. crust is compressed into the crustal root (Houseman et al., 236 The San Andreas Fault in Southern California and Its Relationship to Lithospheric Structure 237 Figure 1. Fault map of southern California and cross sections showing dipping San Andreas fault (SAF). Black and green lines, profiles discussed in paper; green dots, additional locations discussed. Profiles and locations are numbered sequentially from northwest to southeast, 1–15. Cross sections along black lines (1, 4, 5, 8, 10, 12, 13, 14, and 15) are shown as satellite diagrams with geographic location labels. Cross section 10 is reproduced with interpreted SAF (red line) from Jones et al. (1986; their fig. 3a). Cross sections 13–15 are reproduced with interpreted SAF (red lines) from Lin et al. (2007; their fig. 14). Green lines 2, 6, and 9 are locations of seismicity cross sections of Figure 9. Green line 7 is location of seismic-imaging profile through San Bernardino Valley (see text). Green dots 3 and 11 are locations, Three Points and Desert Hot Springs (see text). Heavy red line, San Andreas fault; reddish brown lines, other active faults (Jennings, 1994). Blue body, horizontal slice through upper-mantle high-velocity body at 110-km depth (see Kohler et al., 2003, their fig. 7A). Colors in cross sections 1, 8, and 12 are explained in Figure 3. Colors and symbols in cross sections 4 and 5 are explained in Figure 10. Abbreviations: CV, Coachella Valley; LAB, Los Angeles basin; SGP, San Gorgonio Pass; SS, Salton Sea. 2000; Fuis et al., 2001; Godfrey et al., 2002; Fuis throughout the crust, geophysical observations generally et al., 2007). constrain the SAF geometry in only the upper 5–15 km of the crust. For simplicity, we assume a constant dip as a function of depth for the SAF at the location of every Data Constraining Fault Dip constraint along its trace, except in the San Gorgonio Pass At a number of locations in southern California, we area where shallower indicators suggest a very gentle dip estimate the dip of the SAF from analysis of potential field, but deeper indicators suggest a steeper dip. In addition, seismicity, and seismic-imaging observations (Fig. 1). Where we linearly interpolate these dip values along the SAF trace possible, we assign an uncertainty to the dip values. Except between the constraint locations (Fig. 2), with interpolation for the LARSE observations that constrain the geometry distances that range from about 15 to 120 km. Because of the 238 G. S. Fuis, D. S. Scheirer, V. E. Langenheim, and M. D. Kohler Figure 2. (a) Plan view of dipping SAF model surface colored by depth; gray colors, unconstrained projections. Mountain ranges within Transverse Ranges (see Fig. 1): SGM, San Gabriel Mountains; SBM, San Bernardino Mountains; LSBM, Little San Bernardino Mountains; SAFOD, San Andreas Fault Observatory at Depth. (b) Oblique view of SAF surface from southeast. heterogeneous nature of our constraining observations and section (at Three Points; Fig. 1; Fig. 2a), gravity and because of the simplifications and interpolations required magnetic data also suggest a southwestward dip (30°–75°), to generate this dipping SAF model, we view the strength but uncertainty is greater. In the San Gorgonio Pass area, of this model to be simply its depiction of systematic and approximately 250 km southeast of the Big Bend cross sec- significant dip variations along the SAF. tion, gravity modeling indicates that the Banning strand of the SAF dips very gently (10°–15°) to the northeast in the Potential Field upper few kilometers (Griscom and Jachens, 1990; Langen- heim et al., 2005) (Fig. 2a). In this study we model two A published model of gravity data across the SAF in the vicinity of the Big Bend in the western Transverse Ranges additional profiles of magnetic data, at San Bernardino (Griscom and Jachens, 1990) indicates a southwestward dip and at Indio (Fig. 1, profiles 8 and 12), that indicate dips as shallow as 55° (Fig. 1, profile 1; Fig. 3a). The SAF is mod- of 37° and 65°, respectively (Fig. 3b,c). eled to a depth as great as 5 km. At 120–180 km northwest of A nearly continuous magnetic anomaly extends along this model location (at Cholame and the San Andreas Fault the SAF from the northwestern San Bernardino Mountains Observatory at Depth [SAFOD]); Fig. 2a], a steeper south- to Indio, averaging 200–500 nT at a nominal height of 300 m west dip is seen in the upper few kilometers of the crust, above terrain (Fig. 4; Griscom and Jachens, 1990). This 70°–90°from gravity data at Cholame (Griscom and Jachens, magnetic anomaly is predominantly positive and is spatially 1990) and 83° from drilling observations at SAFOD (Zoback associated with outcrop of units labeled as Precambrian et al., 2010). About 50 km southeast of the Big Bend cross igneous and metamorphic rock complex in the portions of The San Andreas Fault in Southern California and Its Relationship to Lithospheric Structure 239 Figure 3. Block models for three sites along SAF. (a) Model of gravity data at the Big Bend of SAF (Griscom and Jachens, 1990). 3 Location is profile 1 on Figure 1. D, density differences (kg=m ) of upper crustal rocks of North American plate (NAM) compared 3 with Pacific plate (PAC, density 2670 kg=m ). (See also modeling of combined gravity and aeromagnetic data at Big Bend in Appendix A.) (b, c) Block models of magnetic data at San Bernardino and Indio sites on SAF from this study. Locations are profiles 8 and 12 on Figure 1.
Details
-
File Typepdf
-
Upload Time-
-
Content LanguagesEnglish
-
Upload UserAnonymous/Not logged-in
-
File Pages16 Page
-
File Size-