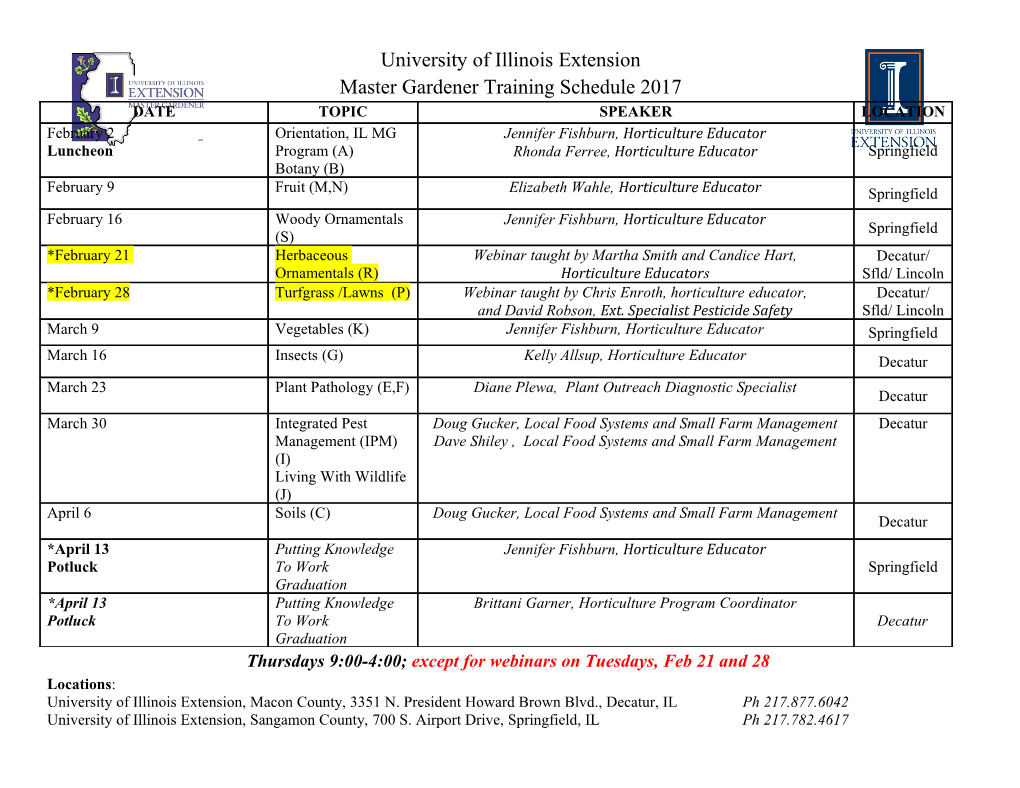
6/26/17 From the Dark Ages to the Cosmic Renaissance 1st BHI Conference Harvard University High-redshift frontier Cambridge, May 2017 (t<1 Gyr) Black Holes in the High-Redshift Universe 1st Stars st Volker Bromm 1 Galaxies Department of Astronomy University of Texas at Austin • First Stars (Pop III) à From Simplicity to Complexity Structure Formation from Cosmological ICs Formation of a Population III Star: (Stacy, Greif & Bromm 2010, MNRAS, 403, 45) à Properties of First Stars, Galaxies, Black Holes • ab initio structure formation (or: the Laplace Demon at work) Minihalo: 6 M ~ 10 Mo Input: Output: R ~ 100 pc Laws of nature Ω , Ω , Ω , B Λ m Stellar IMF, SFE, z ~ 20 σ8, ns, H0, Multiplicity… abundances, (supercomputer) DM particle physics Black holes (Pre-stellar) Core: 3 M ~ 10 Mo ! Non-linear amplifier! R ~ 1 pc 1 6/26/17 How to make a star? Merging of Pop III Protostars (Greif, Bromm, et al. 2012, MNRAS, 424, 399) • Gravitational instability (Jeans 1902) Gas Temperature • Gravity must overwhelm opposing thermal pressure: - AREPO (Springel 2010) Gas (Hydrogen/ - cosmological ICs Need: Helium) - completely ab initio: Mass > NO sink particles! Jeans mass (MJ) Sir James Jeans • Primordial clouds are hotter à need larger masses à first stars were more massive! Population III : Radiative Feedback Pop III Star Formation: Growth by Accretion (Stacy, Greif & Bromm 2012, MNRAS, 422, 290) (Stacy, Greif & Bromm 2012, MNRAS, 422, 290) 16 A. Stacy, T. H. Greif and V. Bromm Stellar mass vs. time age accretion rates over a longer period of time (50,000 yr) First Stars: Growth Under Feedback 13 and found that gravitational and Rayleigh-Taylor instabili- 1,500 yr 2,000 yr 3,000 yr ties would continue to feed mass onto the disk and stars. We also note that, despite their very similar numeri- nocal setups, feedbackthe disk evolution and accretion rate of our ‘no- feedback’ case differs somewhat from that described in Stacy primary et al. (2010). Several distinctions between the simulations, however, explain this. The high-density cooling and chem- istry is updated from that used in Stacy et al. 2010 (see Section 2.2). We also use an adaptive softening length in- stead of a single softening length for all gas particles as in secondary Stacy et al. (2010), and our criteria for sink accretion are slightly more stringent. The main contribution to the dif- ference, however, is likely the stochastic nature of the sink particle dynamics. While no sink was ejected in Stacy et al. (2010), the sink ejection and subsequent rapid velocity of the main sink in our ‘no-feedback’ case altered the disk struc- ture, and the main sink would likely have grown to a higher mass otherwise. Nevertheless, the final sink masses in both Figure 7. Projected ionization structure of gas at 1500, 2000, and 3000 yr after initial sink formation. White lines depict the density simulations were still the same to within a factor of two. 40,000 AU Figure 10. Effect of radiative feedback on protostellar accretion. 7.5 8 8.5 9 −3 - a dominant binary has formed (~ 20 and ~10 MO) afterThe radiativ~5,000e feedbac yrk seen here is much stronger than contours of the disk at densities of 10 , 10 , 10 , and 10 cm . Box length is 40,000 AU. Note the pronounced hour-glass morphology Black solid line shows mass growth with no radiative feedback, ii the analytical prediction of McKee & Tan (2008), even when of the developing ultra-compact H region, roughly perpendicular to the disk. This structure gradually expands and dissipates the disk of accretion while black dotted line shows the ‘with-feedback’ case. The blue gas from above and below, reducing the scale height of the disk. solid line is a double powerlaw fit to the sink growth rate for the considering the combined accretion rate of both sinks. They ‘no-feedback’ case, and the red dotted line is a double powerlaw found that a Pop III star could grow to over 100 M⊙ through 4 - Similar mass fitrangefor ‘with-feedbac foundk’ case. in TheHosokawadashed line shows etthe al.growth 2011disk (Science)accretion, as disk shadowing allowed mass to flow onto - after ~5,000 yr: UCHII Region with r~10 AU of the second-most massive sink. Radiative feedback along with the star even while the polar regions became ionized. Our fragmentation-induced starvation leads to a divergence in the ac- lack of resolution prevents this disk shadowing from being cretion histories in less than 1000 yr, and in the ‘with-feedback’ fully modeled on sub-sink scales, and the ionizing photon case the main sink does not grow beyond ∼ 20 M⊙ in the time emission emanating from the sink edge is likely overesti- of the simulation. mated along some lines-of-site. This especially applies to angles just above and below the disk that quickly became ionized in our ‘with-feedback case’, when in reality these cent work by Peters et al. (2010b). They similarly examine regions are unlikely to become ionized until sometime later, ionizing and non-ionizing radiative feedback on massive star only after the gas that is polar the disk becomes ionized first. formation, though they study the case of present-day star McKee & Tan (2008) furthermore assumed disk axisymme- formation, and their initial configuration was different from try, which does not describe the disk in either of our test ours in that they began with a 1000 M⊙ rotating molecular cases. Nevertheless, our shielding prescription does indeed cloud core. They find H ii regions which fluctuate in size and keep the disk from becoming ionized in our ‘with-feedback’ shape as gas flows onto the stars, and that the final mass case. However, the I-front does not expand in a perfectly uni- of the largest stars is set by ‘fragmentation-induced star- form fashion along the polar directions, as the disk rotates, vation,’ a process in which the smaller stars accrete mass and the position of the main sink within the disk varies as flowing through the disk before it is able to reach the most it orbits its companion sink. Thus, different angles will be Figure 8. Projected H2 fraction of gas at 1500, 2000, and 3000 yr after initial sink formation. Gray lines depict the density contours massive star. This is in contrast to models in which the final shielded at different times. Once gas along a certain direction 7.5 8 8.5 9 −3 of the disk at densities of 10 , 10 , 10 , and 10 cm . Box length is 40,000 AU. Note how the morphology of the neutral region is stellar mass is set once ionizing radiation shuts off the disk has been ionized, it may recombine at later times, but LW similar to that of the ionized region. The molecular inflow gradually becomes confined to the disk plane. accretion (e.g. McKee & Tan 2008). In our ‘with-feedback’ radiation prevents most of this gas from cooling back down case we find that fragmentation-induced starvation occurs to below a few thousand Kelvin. The pressure wave of between 1000 and 2000 yr, while afterwards radiative feed- warm neutral gas sourced LW feedback, along with ∼ front is shown in Figure 7, where we can see that the I-front where cs is the ionized gas soundspeed and xs is the position back does lead to a further decline in the sink accretion rate an extended region of neutral gas sourced by for- expands as an hour-glass shape above and below the disk. of the shock in similarity coordinates, which for our case is and the disk mass. Soon after the I-front breaks out from merly ionized particles that recombined, leads to a bubble of neutral gas that continues to expand in all As the I-front expands and widens to encompass a larger xs = 2.54 (see panel b of Figure 9). the sink, the second largest sink accretes much more quickly angular region, it dissipates the more diffuse disk gas above Multiple factors cause deviations from the analytical than the first, intercepting a large portion of infalling mass directions except within a few degrees of the disk plane, and mass flow onto the disk and sinks is greatly reduced. and below the mid-plane, leading to a decline in the disk solution. For instance, the density structure of the gas is that otherwise would be accreted by the main sink. Indeed, the final estimated mass of the main sink is similar to that Thus, while our results underestimate the effect of2 shielding, scale height. Figure 9 compares the I-front evolution with not spherically symmetric. Furthermore, the initially close found in Peters et al. (2010a), where they found the combi- it still highlights how non-axisymmetry will enhance the ef- that predicted by the analytical Shu champagne flow solu- proximity of the ionization front to the sink causes the grav- nation of disk fragmentation and radiative feedback led to a fects of radiative feedback, and the true physical case likely tion (Shu et al. 2002, see also Alvarez et al. 2006). Different ity of the sink to have a non-negligible effect on the early most-massive star of 25 M⊙. However, radiative feedback be- lies somewhere in between our ‘with-feedback’ case and the analytical solutions can be found for the evolution of gas H ii region dynamics, a factor that is neglected in the an- comes important after 2000 yr as it eventually prevents mass prediction of McKee & Tan (2008). In a similar vein, non- under the propagation of an I-front into a powerlaw density alytical solution.
Details
-
File Typepdf
-
Upload Time-
-
Content LanguagesEnglish
-
Upload UserAnonymous/Not logged-in
-
File Pages7 Page
-
File Size-