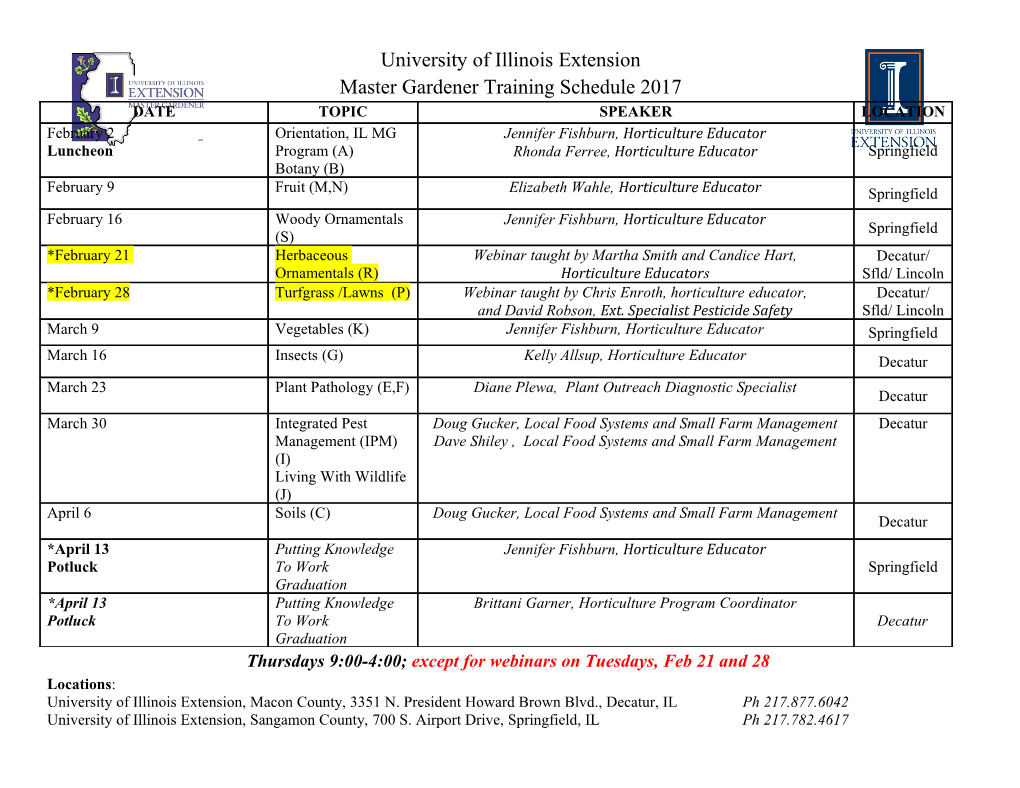
Proceedings of 7th Transport Research Arena TRA 2018, April 16-19, 2018, Vienna, Austria Hydrogen Production and Storage by Oxidation and Reduction of Iron-based Oxygen Carriers Sebastian Bocka, Robert Zachariasa, Richard Schauperlb, Jürgen Rechbergerb, c c c a* Florian von Hofen , Gernot Voitic , Uwe Strohmeyer , Viktor Hacker a Institute for Chemical and Environmental Engineering, Graz University of Technology, Inffeldgasse 25C, 8010 Graz, Austria b AVL List GmbH, Hans List Platz 1, 8020 Graz, Austria c Rouge H2 Engineering GmbH, Reininghausstraße 13, 8020 Graz, Austria Abstract The fixed-bed chemical-looping hydrogen (CLH) process offers the possibility of decentralised small-scale hydrogen production and storage from local available renewable hydrocarbon sources like biogas. The detailed process simulation indicated the ability of converting a broad range of different bio-based feedstocks into hydrogen. Experiments for hydrogen production in a lab-scale reactor showed the ability to release high-pressurized hydrogen with up to 50 bar from the fixed-bed production system without an additional gas compression step. Hydrogen with a purity up to 99.999% was produced in the performed lab-scale experiments to meet the stringent requirements for FCEVs. Detailed process simulations for combined loading and unloading of the oxygen carrier with a typical biogas feedstock are presented. The thermodynamic analyses revealed a high influence of the reformer synthesis gas composition and also of the internal recirculation on the overall process efficiency. The previously loaded oxygen carrier is appropriate as process-integrated storage solution to cover fluctuating demands during the day i.e. for use in fuel stations. A long-term storage and distribution is possible due to the easy handling and the very high volumetric storage capacity compared to conventional tube trailer transportation. Keywords: Hydrogen production; hydrogen storage; chemical looping hydrogen; steam-iron process. _________________________________ *Viktor Hacker, Sebastian Bock. Tel.: +43-316-873-8781; fax: +43-316-8738782. E-mail address: [email protected], [email protected] 1 Viktor Hacker, Sebastian Bock / TRA2018, Vienna, Austria, April 16-19, 2018 Nomenclature FCEV Fuel cell electric vehicle GC Gas chromatography LHV Lower heating value MFC Mass flow controller n Molar amount S/C Steam to carbon ratio 1. Introduction Dealing with the earth’s limited natural resources and preserving the liveable environment are main challenges of our time. Beside political discussions, a broad consensus in the scientific community for a human-caused temperature rise in the atmosphere exists. However, the considerable increase of carbon emissions since the start of industrialisation in the late 19th century (Fig. 1) already caused the rise of the carbon dioxide content in the atmosphere. It is our responsibility to show pathways for a sustainable energy system, based on the high efficient utilisation of renewable energies. Fig. 1:Annual global anthropogenic carbon dioxide emissions (Gt CO2-equivalent per year) from fossil fuel combustion, cement production and flaring, and forestry and other land use (FOLU) 1750–2011, [IPCC, Climate Change 2014 Synthesis Report, Fifth Assessment Report, Geneva, 2014]. Fuel cell electric vehicles (FCEVs) are technical mature and offer high-efficient transport solutions. A wide distribution of this technology is primarily inhibited by the high price of the available vehicles as well as by lacking infrastructure for refuelling the compressed hydrogen. In addition, fuel cell vehicles demand high standards of hydrogen fuel purity to inhibit degradation of the used catalysts in low temperature fuel cells. A commercial standard for PEM-fuel cells is a hydrogen purity above 5.0, with the sum of all contaminants being lower than 0.001% (10 ppm). Since steam reforming of various fossil hydrocarbon feedstocks is the basis for more than 90% of present-day hydrogen production, fuel cell applications just displace the location of carbon dioxide emissions to the fuel producer. The sustainability of hydrogen as a secondary energy carrier largely depends on the feedstock, the production method and the logistical efforts for the whole allocation process. Great efforts have been made over the last decades to exhibit potential solutions for hydrogen generation from renewable energy sources. 1.1. Chemical Looping Hydrogen Production and Storage Chemical-looping combustion is a versatile process to use metal-based oxides as oxygen carriers for combined reduction and oxidation processes. It utilises the oxygen carrier material to exchange oxygen atoms between gaseous phases in several consecutive steps. This technology is proposed primarily for the combustion reaction of various feedstocks with inherent carbon dioxide sequestration. In a separate air reactor, the oxygen carrier is regenerated and heat is released. The steam-iron process uses the same principle (Hacker et al., 2017): an iron- based oxygen carrier is reduced by hydrocarbon feedstocks to obtain iron from iron oxides. Afterwards steam is used to oxidize the iron to magnetite and pure hydrogen is released (Fig. 2). 2 Viktor Hacker, Sebastian Bock / TRA2018, Vienna, Austria, April 16-19, 2018 Fig. 2: Oxidation and reduction reactions for hydrogen production by the steam iron process. Hacker et al. (2003) proposed the Reformer sponge iron cycle (RESC) as combined process for the reforming of hydrocarbons and hydrogen generation with the steam-iron process. Several renewable hydrocarbons like bioethanol, landfill gas, biogas or syngas from biomass gasification can be utilised as feedstocks. The process design allows the implementation of a small-scale decentralised hydrogen production from local available renewables or fossil hydrocarbons. In addition, hydrogen can be directly stored in the production system as iron, enabling an on-demand release of pure hydrogen through the addition of steam. Therefore, fluctuating quantities of bio-based feedstocks from the respective production method as well as a varying demand can be balanced by the integrated storage mechanism. In the FFG-project “HyStORM – Hydrogen Storage by Oxidation and Reduction of Metals” the feasibility of in- situ hydrogen storage as reduced pure iron and allocation of hydrogen by oxidising the oxygen carrier is evaluated. The process design is analysed regarding the utilisation of different renewables as feedstock. In addition, the release of compressed hydrogen is possible if the reactor system allows a pressurised operation in oxidation step. The process-integrated pressurisation would facilitate the compression of hydrogen to 700 bar, as demanded for mobility applications. 2.1. High pressure fixed-bed reactor A small-scale reactor system is used to evaluate the steam-iron process step in a lab plant (Fig. 3) as published by Voitic et al. (2016). The inner reactor diameter di is 15.26 mm and it is heated by an external electric furnace. The oxygen carrier is situated in a high-pressure reactor which is operated in fixed-bed mode. A thermocouple inside the oxygen carrier bed is used to control the process temperature with an outside electrical furnace heating the reactor system. To evaluate different syngas compositions from an upstream reformer, gases are mixed with several mass flow controllers for the reduction cycle. The oxidation of the fixed bed oxygen carrier bed is carried out by feeding ultrapure water by a high-pressure pump. Pressure built-up is achieved by (partially) closing the outlet mass flow controller (MFC). Water is condensed before the outlet MFC and an additional drying tube protects the mass flow controller from condensed water. The outlet gas composition is periodically monitored with a Micro-GC gas analyser (Inficon Micro-GC 3000), the overall outlet gas flow is determined with an inert gas as external standard. 3 Viktor Hacker, Sebastian Bock / TRA2018, Vienna, Austria, April 16-19, 2018 Fig. 3: Experimental setup of lab-scale reactor for high-pressure hydrogen production, Voitic et. al. (2016). 2.2. Thermodynamic model Several thermodynamic process evaluations were carried out to investigate feasible process parameters. They include a full thermodynamic simulation of both reduction and oxidation process, an additional flue gas burner and a heat exchanger to determine the additional net energy demand for process heating (Fig. 4). From this model, an overall process efficiency can be calculated by comparing the produced hydrogen LHV to the overall feedstock LHV (1). The thermodynamic gas equilibria of the oxidation stages can be depicted from the Baur-Glaessner diagram for the magnetite-wuestite (Fe3O4-FeO) and wuestite-iron (FeO-Fe) step for different temperatures. Fraser et al. (2006) varied two main process parameters for the simulation: First, the process temperature, which is influencing the thermodynamic equilibrium of the oxygen carrier material and second, a variation of the recycle rate for the recycle stream was evaluated (2). 퐿퐻푉퐻2 ∙ 푛퐻2 휂푡ℎ = (1) 퐿퐻푉퐹푒푒푑 ∙ 푛퐹푒푒푑+퐿퐻푉퐻푒푎푡푒푟 ∙ 푛퐻푒푎푡푒푟 푛푟푒푐푦푐푙푒 푟푒푐푦푐푙푒 푟푎푡푒 = (2) 푛푟푒푎푐푡표푟 Superheater Reformer Reactor Lean gas burner Cooler Biogas, Flue gas H2O Air Lean gas recycle stream Superheater Reactor Condenser H2, H2O(l) H2O Fig. 4: Simplified flowsheet of the reformer steam-iron process for evaluation purposes. 4 Viktor Hacker, Sebastian Bock / TRA2018, Vienna, Austria, April 16-19, 2018 Extensive research has been conducted in the last years to improve
Details
-
File Typepdf
-
Upload Time-
-
Content LanguagesEnglish
-
Upload UserAnonymous/Not logged-in
-
File Pages6 Page
-
File Size-