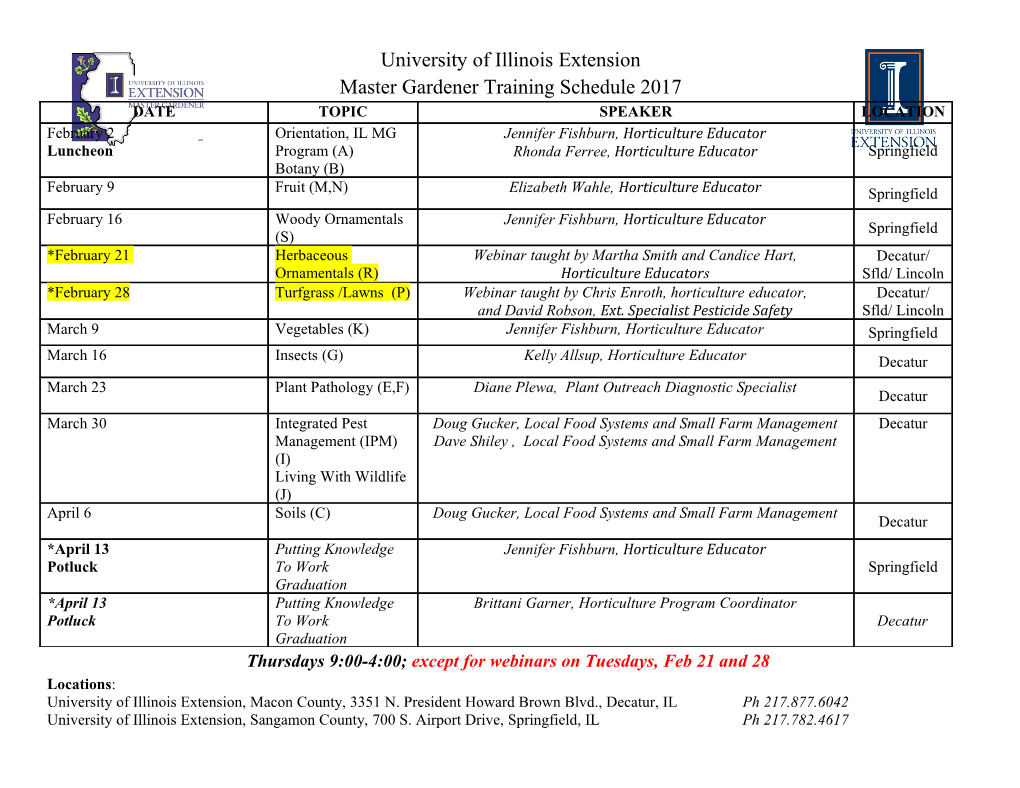
Introduction to Supersymmetry(1) J.N. Tavares Dep. Matem¶aticaPura, Faculdade de Ci^encias,U. Porto, 4000 Porto TQFT Club 1Esta ¶euma vers~aoprovis¶oria,incompleta, para uso exclusivo nas sess~oesde trabalho do TQFT club CONTENTS 1 Contents 1 Supersymmetry in Quantum Mechanics 2 1.1 The Supersymmetric Oscillator . 2 1.2 Witten Index . 4 1.3 A fundamental example: The Laplacian on forms . 7 1.4 Witten's proof of Morse Inequalities . 8 2 Supergeometry and Supersymmetry 13 2.1 Field Theory. A quick review . 13 2.2 SuperEuclidean Space . 17 2.3 Reality Conditions . 18 2.4 Supersmooth functions . 18 2.5 Supermanifolds . 21 2.6 Lie Superalgebras . 21 2.7 Super Lie groups . 26 2.8 Rigid Superspace . 27 2.9 Covariant Derivatives . 30 3 APPENDIX. Cli®ord Algebras and Spin Groups 31 3.1 Cli®ord Algebras . 31 Motivation. Cli®ord maps . 31 Cli®ord Algebras . 33 Involutions in V .................................. 35 Representations . 36 3.2 Pin and Spin groups . 43 3.3 Spin Representations . 47 3.4 U(2), spinors and almost complex structures . 49 3.5 Spinc(4)...................................... 50 Chiral Operator. Self Duality . 51 2 1 Supersymmetry in Quantum Mechanics 1.1 The Supersymmetric Oscillator i As we will see later the \hermitian supercharges" Q®, in the N extended SuperPoincar¶eLie Algebra obey the anticommutation relations: i j m ij fQ®;Q¯g = 2(γ C)®¯± Pm (1.1) m where ®; ¯ are \spinor" indices, i; j 2 f1; ¢ ¢ ¢ ;Ng \internal" indices and (γ C)®¯ a bilinear form in the spinor indices ®; ¯. When specialized to 0-space dimensions ((1+0)-spacetime), then since P0 = H, relations (1.1) take the form (with a little change in notations): fQi;Qjg = 2±ij H (1.2) with N \Hermitian charges" Qi; i = 1; ¢ ¢ ¢ ;N. Let us see some imediate consequences of relations (1.2: ² The supercharges Qi are constants of motion. In fact: [H; Q] = [Q2;Q] = 0 (1.3) where Q is any of the Qi. ² The Hamiltonian H is an hermitian positive operator, and so the energy spectrum is always positive de¯nite. In fact: 2 2 H = Q1 = ¢ ¢ ¢ = QN (1.4) So, 8jÃi 2 H we have: hÃjHjÃi = hÃjQ2jÃi = hÃjQyQjÃi = kQjÃik2 ¸ 0 where Q is any of the Qi. This also proves that: ² ker H = \i ker Qi (1.5) Since the Hamiltonian H is a positive operator, any eigenstate jÃ0 > of H with zero eigenvalue is a \ground state", and for such a ground state we have that QijÃ0 >= 0; 8i. We then say that the \supersymmetry is unbroken". When there is no eigenstate with zero eigenvalue, then the ground state jÃ0i has energy EÃ0 > 0. This implies that QjÃ0i 6= 0 and we then say that we have \spontaneous susy breaking". Now we focus our attention in the N = 2 model, which we call the: 1.1 The Supersymmetric Oscillator 3 \Supersymmetric Oscillator" In this case let us de¯ne the following two \nonhermitian supercharges", adjoint of each other: def 1 S = (Q + iQ ) 2 1 2 def 1 S = Sy = = (Q ¡ iQ ) (1.6) 2 1 2 Then we have the following \representation" for the above (N = 2)-Susy algebra: 2 2 H = Q1 = Q2 = fS; Sg S2 = S2 = 0 (1.7) We also have [H; Q] = 0, where Q is any of the Qi;S or S. Consider the Hilbert space H with basis: jnB; nF i nB = 0; 1; 2; ¢ ¢ ¢ ; 1 nF = 0; 1 (1.8) y where nB and nF are \boson" and \fermion occupation numbers" respectivelly, and let a; a \anihilation-creation" bosonic operators, and f; f y \anihilation-creation" fermionic opera- tors, acting on H in the standard way. They satisfy the following commutation and anti- commutation relations: [a; ay] = 1 ff; f yg = 1 f 2 = (f y)2 = 0 [a; f] = 0 (1.9) Then if we put: def S = k af y \destroy a boson ­ create a fermion" def S = k ayf \create a boson ­ destroy a fermion" (1.10) where k is a constant so that S and S are adjoints of each other (S = Sy), we see that: y S jnB; nF i = k af jnB; nF > / jnB ¡ 1; nF + 1i y S jnB; nF i = k a fjnB; nF > / jnB + 1; nF ¡ 1i (1.11) so that these operators convert a boson into a fermion and vice-versa. Moreover we can verify properties (1.7), using (1.9). 1.2 Witten Index 4 Now what about the Hamiltonian? We compute: H = fS; Sg = k2(af yayf + ayfaf y) 1 1 = k2(aya + ) + k2(f yf ¡ ) 2 2 def = HB + HF (1.12) So the H is the sum of two non-interacting terms: the Hamiltonian of the bosonic oscillator HB with energy spectrum EB, and the Hamiltonian of the fermionic oscillator HF with energy spectrum EF given respectivelly by: 1 1 H = k2(aya + ) E = k2(n + ) n = 0; 1; 2; 3; ¢ ¢ ¢ B 2 B B 2 B 1 1 H = k2(f yf ¡ ) E = k2(n ¡ ) n = 0; 1 (1.13) F 2 F F 2 B Note that: 2 y y y y y nF = f f f f = f ff; f gf = f f = nF and so in fact the eigenvalues of nF are 0; 1 which is the \Pauli exclusion principle". Note also that the frequencies ! = k2 of these two oscillators are the same. 1.2 Witten Index For the above (N = 2)-Susy QM model, we can de¯ne an operator: def (¡1)F = (¡1)nF 1l such that: F F 2 F y F f(¡1) ;Qig = 0 ((¡1) ) = 1l ((¡1) ) = (¡1) (1.14) Converselly, given an Hilbert space H and hermitian operators H; Q; (¡1)F such that (¡1)F is bounded and: H = Q2 ((¡1)F )2 = 1l fQ; (¡1)F g = 0 (1.15) we can de¯ne a (N = 2)-Susy QM model by putting: F Q1 = Q and Q2 = i(¡1) Q We explore now the abstract data given by an Hilbert space H and hermitian operators H; Q; (¡1)F , with (¡1)F bounded, and verifying conditions (1.15). Here follows some properties of this \abstract Susy model", fH; Q; (¡1)F g, which are imediate consequences of conditions (1.15): 1.2 Witten Index 5 I ² [(¡1)F ;H] = [(¡1)F ;Q2] = f(¡1)F ;QgQ ¡ QfQ; (¡1)F g = 0 (1.16) II ² We have a decomposition of H in eigenspaces of (¡1)F corresponding to the eigenvalues §: H = Hb © Hf with: F Hb = f à 2 H :(¡1) à = +à g F Hf = f à 2 H :(¡1) à = ¡Ã g (1.17) F so that (¡1) acts on H as: " # 1l 0 (¡1)F = b 0 ¡1lf III ² The involution (¡1)F induces also a decomposition on the algebra of operators acting on H. If: " # AB K = CD acts on H = Hb © Hf , then: " # A 0 - K is \bosonic" or \even" i® [(¡1)F ;K] = 0 i® K = 0 D " # 0 B - K is \fermionic" or \odd" i® f(¡1)F ;Kg = 0 i® K = C 0 IV ² In particular, since Q is hermitian and anticommutes with (¡1)F , we have that Q is odd and: " # 0 Ay Q = (1.18) A 0 So, applying Q to a vector à = Ãb © Ãf 2 H, we have: " #" # " # 0 Ay à Ayà Qà = b = f A 0 Ãf AÃb and since this belongs to Hb © Hf we must have: QbHb = A : Hb ¡! Hf y QbHf = A : Hf ¡! Hb (1.19) Note also that: " # AyA 0 H = (1.20) 0 AAy 1.2 Witten Index 6 V ² Now we turn to the fundamental property of this Susy model. Let à be an eigenvalue of H with positive energy E > 0: Hà = Eà E > 0 Then, as [H; Q] = 0 we have: H(QÃ) = Q(HÃ) = E (QÃ) which means that Qà is again an eigenvalue of H with the same positive energy E > 0. Note that if E = 0 we can not apply this reasoning, since Hà = 0 implies that: 0 = hÃjHjÃi = hÃjQ2jÃi = hÃjQyQjÃi = kQÃk2 and so Qà = 0 which is not an eigenvector. As we have seen, if à 2 Hb (resp., Hf ) then Qà 2 Hf (resp., Hb) (we call Qà the \superpartner" of Ã), and so we conclude that \all eigenstates with energy E > 0 are paired": dim ker[(H ¡ E)bHb)] = dim ker[(H ¡ E)bHf )] 8E > 0 (1.21) H Q H E > 0 b Ã! f Bosonic sector Fermionic sector ............................................... Hb E = 0 Hf Nf Nb | {z } Q = 0 here Here we have put: Nb = dim ker(HbHb) Nf = dim ker(HbHf ) (1.22) If either Nb or Nf are nonzero, then there exists a state of zero energy (a ground sate) and supersymmetry is unbroken. So if we can compute Nb or Nf we can decide about Susy breaking. In general this is a di±cult problem, and the only thing available is the di®erence Nb ¡ Nf . Thus we de¯ne the \Witten index" as: ¢W = Nb ¡ Nf (1.23) 1.3 A fundamental example: The Laplacian on forms 7 This has remarkable stability properties. In fact \small perturbations" of the system don't a®ect ¢W , since the states of non-zero energy move always in Bose-Fermi pairs. " # 0 Ay Since Q has the form (1.18), i.e., Q = , with A an elliptic operator, then by A 0 (1.19), we have that: ¢W = Nb ¡ Nf = dim ker A ¡ dim ker Ay = index (A) (1.24) 1.3 A fundamental example: The Laplacian on forms Assume that M is a compact oriented closed smooth n-dimensional Riemannian manifold, and let ­k(M) be the Hilbert space obtained by completion of the space of smooth k-forms, with respect to the usual inner product: Z < ®; ¯ >= ® ^ ¤¯ M Now we construct an \abstract Susy model" fH; Q; (¡1)F g, on the Hilbert space: def n k H = ©k=0 ­ (M) (1.25) by de¯ning: ² H = ¢ = dd¤ + d¤d, the operator closure of the usual laplacian on smooth forms.
Details
-
File Typepdf
-
Upload Time-
-
Content LanguagesEnglish
-
Upload UserAnonymous/Not logged-in
-
File Pages54 Page
-
File Size-