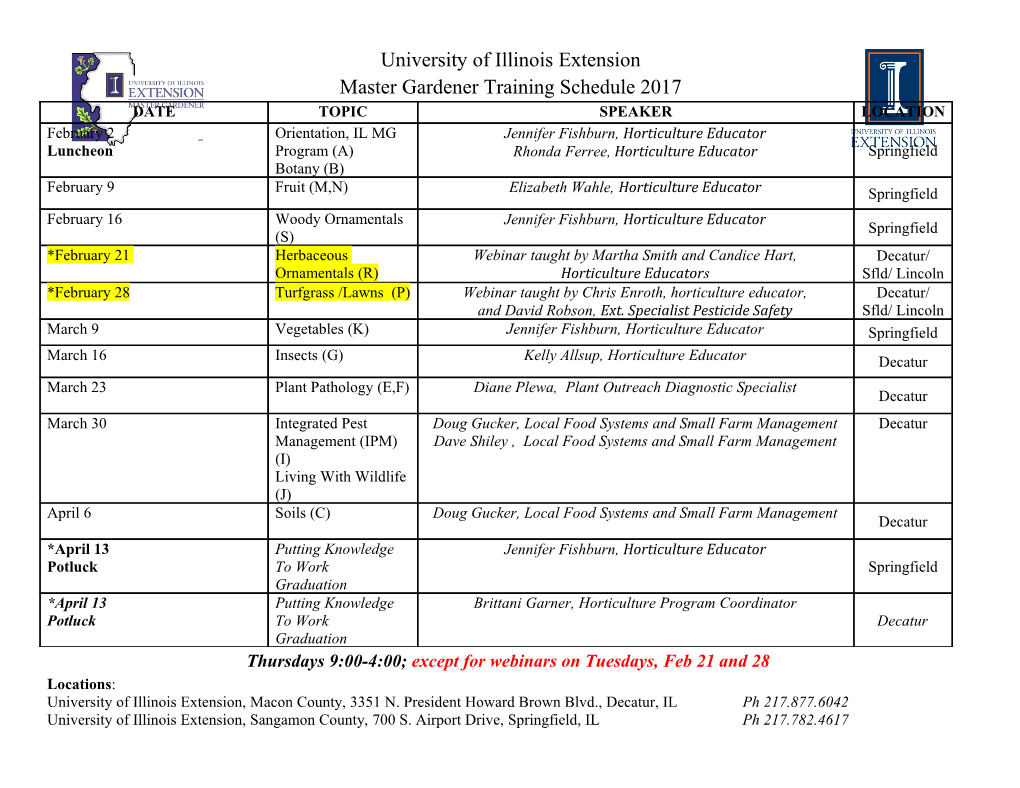
The DNA ejection process in bacteriophage λ Thesis by Paul Grayson In Partial Fulfillment of the Requirements for the Degree of Doctor of Philosophy California Institute of Technology Pasadena, California 2007 (Submitted May 24, 2007) Abstract Bacteriophages have long served as model systems through which the nature of life may be explored. From a physical or mechanical point of view, phages are excellent examples of natural nanotechnology: they are nanometer-scale systems which depend critically on forces, pressures, velocities, and other fundamen- tally physical quantities for their biological functions. The study of the physical properties of phages has therefore provided an arena for application of physics to biology. In particular, recent studies of the motor responsible for packaging a phage gnome into a capsid showed a buildup of pressure within the capsid of tens of atmospheres. This thesis reports a combined theoretical and experimental study on various aspects of the genome ejection process, so that a comparison may be drawn with the packaging experiments. In partic- ular, we examine various theoretical models of the forces within a phage capsid, deriving formulas both for the force driving genome ejection and for the velocity at which the genome is translocated into a host cell. We describe an experiment in which the force was measured as a function of the amount of genome within the phage capsid, and another where the genome ejection velocity was measured for single phages under the microscope. We make direct quantitative comparisons between the theory and experiments, stringently testing the extent to which we are able to model the genome ejection process. i Contents Abstract i 1 Introduction 1 1.1 Phages as an arena for science . 1 1.1.1 The central position of phages in biology . 1 1.1.2 Phages as a focus of physical research in biology . 3 1.2 The phage ejection problem: how does the DNA get out? . 6 2 Models of bacteriophage λ DNA ejection. 7 2.1 Theoretical model of internal pressure . 8 2.1.1 Goals for the model . 10 2.1.2 Simple model I: counterion entropy . 11 2.1.3 Simple model II: empirical interstrand forces . 14 2.1.4 Simple model III: only bending energy . 16 2.1.5 Comparison of the models . 19 2.2 Modeling the mobility of DNA during ejection . 21 2.2.1 Friction in the phage tail . 21 2.2.2 Friction in the phage head . 23 2.2.3 Friction from layer sticking (Grosberg/Rubinstein model) . 24 2.3 Calculating drag forces in the toilet-flush model . 27 2.3.1 Precise definition of osmotic pressure . 27 2.3.2 Implications of the toilet-flush model . 29 ii 3 Experiments on the bacteriophage ejection process 33 3.1 Experimental measurement of ejection force . 33 3.1.1 Results . 35 3.1.2 Error analysis . 39 3.1.3 Discussion . 42 3.2 Real-time in vitro measurement of DNA ejection . 44 3.2.1 Bulk measurements . 45 3.2.2 Single-molecule measurements . 52 3.2.3 Image processing for DNA length determination . 52 3.2.4 The effect of flow on the DNA length . 56 3.2.5 Length calibration . 59 3.2.6 Results . 60 3.2.7 Error analysis . 70 3.3 Real-time in vivo measurement of DNA ejection . 72 3.3.1 Results . 73 3.3.2 Discussion . 76 3.4 Comparison to real-time experiments on DNA packaging . 77 3.4.1 Optical-tweezer measurements on DNA packaging: φ29, λ, and T4. 77 3.4.2 Viscosity effects on packaging . 77 3.4.3 Osmotic pressure effects on packaging . 79 3.4.4 Quantitative disagreement between theory and experiment for φ29 . 80 4 Implications and future work 82 5 Acknowledgments 85 5.1 People . 85 5.1.1 Collaborators . 85 5.1.2 Helpful Discussions . 86 5.2 Help with materials and facilities . 88 5.2.1 Strains . 88 5.2.2 Laboratory space and materials . 88 iii 5.3 Funding . 88 A Glossary 89 B Mathematical variables 90 C Protocols 91 C.1 Bacteriophage propagation . 92 C.1.1 Background . 92 C.1.2 Materials . 92 C.1.3 Procedure . 92 C.2 Extraction of phage DNA . 93 C.2.1 Background . 93 C.2.2 Materials . 93 C.2.3 Procedure . 93 C.3 Field inversion gel electrophoresis . 94 C.3.1 Procedure . 94 C.4 Growing cells on agarose pads . 95 C.4.1 Materials . 95 C.4.2 Procedure . 95 C.5 Lambda plate lysis . 96 C.5.1 Supplies . 96 C.5.2 Procedure . 96 C.6 Lambda plate lysis plates . 97 C.7 Osmotic pressure inhibition assay . 98 C.7.1 Materials . 98 C.7.2 Procedure . 98 C.8 Purification of LamB . 100 C.8.1 Background . 100 C.8.2 Materials . 100 C.8.3 Procedure . 101 C.9 Purification of λ .........................................103 iv C.9.1 Supplies . 103 C.9.2 Differential sedimentation . 103 C.9.3 CsCl gradient . 103 C.10 Real-time in vitro bulk ejection . 105 C.10.1 Materials . 105 C.10.2 Procedure . 105 C.11 Real-time in vitro single molecule ejection . 106 C.11.1 Initial preparation upstairs . 106 C.11.2 Downstairs at the microscope . 107 C.12 Real-time in vivo single molecule ejection . 111 C.12.1 Materials . 111 C.12.2 Procedure . 111 C.13 TM buffer . 113 C.13.1 Procedure (10x) . 113 C.14 Tethering DNA fragments . 114 C.14.1 Materials . 114 C.14.2 Preparing labeled λ-bio DNA stock . 114 C.14.3 Cutting the DNA . ..
Details
-
File Typepdf
-
Upload Time-
-
Content LanguagesEnglish
-
Upload UserAnonymous/Not logged-in
-
File Pages133 Page
-
File Size-