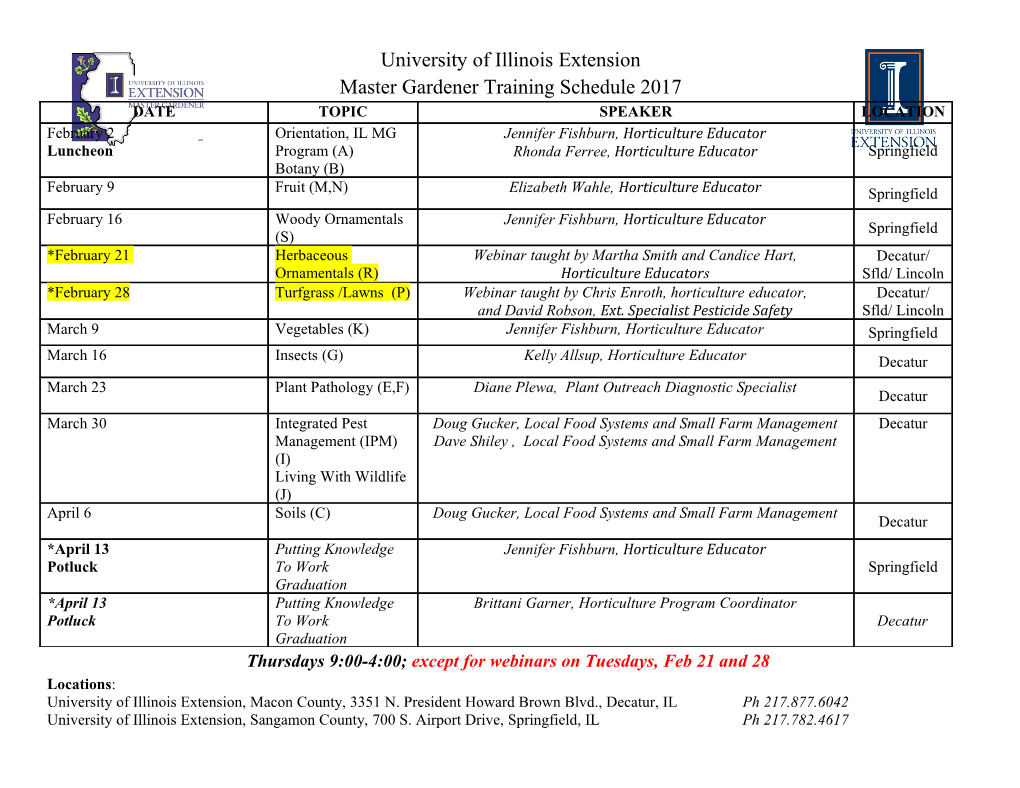
Molecular Aspects of Medicine 28 (2007) 4–41 www.elsevier.com/locate/mam Review Suicide genes for cancer therapy Daniel Portsmouth a,b, Juraj Hlavaty a, Matthias Renner c,* a Research Institute for Virology and Biomedicine, University of Veterinary Medicine, Vienna, Austria b Christian-Doppler Laboratory for Gene Therapeutic Vector Development, Vienna, Austria c Austrianova Biotechnology GmbH, Veterinaerplatz 1, A-1210 Vienna, Austria Received 15 December 2006; accepted 18 December 2006 Abstract The principle of using suicide genes for gene directed enzyme prodrug therapy (GDEPT) of cancer has gained increasing significance during the 20 years since its inception. The astute application of suitable GDEPT systems should permit tumour ablation in the absence of off-target toxicity commonly associated with classical chemotherapy, a hypothesis which is supported by encouraging results in a multitude of pre-clinical animal models. This review provides a clear explanation of the rationale behind the GDEPT principle, outlining the advantages and limitations of different GDEPT strategies with respect to the roles of the bystander effect, the immune system and the selectivity of the activated prodrug in contribut- ing to their therapeutic efficacy. An in-depth analysis of the most widely used suicide gene/pro- drug combinations is presented, including details of the latest advances in enzyme and prodrug optimisation and results from the most recent clinical trials. Ó 2007 Elsevier Ltd. All rights reserved. Keywords: Gene therapy; Gene directed enzyme prodrug therapy; Suicide gene; Thymidine kinase; Cyto- sine deaminase; Cytochrome P450; Purine nucleoside phosphorylase; Carboxypeptidase G2; Nitro- reductase; Bystander effect * Corresponding author. Tel.: +43 1 25077 2301; fax: +43 1 25077 2390. E-mail address: [email protected] (M. Renner). 0098-2997/$ - see front matter Ó 2007 Elsevier Ltd. All rights reserved. doi:10.1016/j.mam.2006.12.001 D. Portsmouth et al. / Molecular Aspects of Medicine 28 (2007) 4–41 5 Contents 1. Introduction . ............................................... 5 2. Thymidine kinase ............................................... 8 3. Cytosine deaminase . ................. 14 4. Cytochrome P450/cytochrome P450 reductase. ................. 17 5. Nitroreductase . ................. 21 6. Carboxypeptidase G2 . ................. 22 7. Purine nucleoside phosphorylase . ................. 23 8. Conclusion . ................. 24 References. ................. 25 1. Introduction Classical chemotherapy has been established over the course of several decades as a standard treatment for cancer patients, proving successful in retarding a vari- ety of tumour types (Chabner and Roberts, 2005). Since currently available drugs are not cancer specific, however, difficulties remain in attaining therapeutic intratu- moral concentrations in the absence of systemic and off-target organ toxicity, meaning that their therapeutic index is often poor (Chatelut et al., 2003; Scripture and Figg, 2006). This is particularly the case in the treatment of solid tumours, in which poor neo-vascularisation and associated necrotic regions often render them relatively refractory to the efficient uptake of systemically delivered drugs (Jain, 2001, 2005; Munn, 2003). Decades of cancer research, however, have provided detailed knowledge of the molecular mechanisms involved in tumorigenesis, tumour invasion, angiogenesis and metastasis, as well as those involved in tumour suppression, growth control, apoptosis and the immune response (Kufe et al., 2005). This knowledge has in turn provided targets for cancer gene therapy (Seth, 2005) and cell based therapy (CBT) (Gunzburg and Salmons, 2005; Pereboeva and Curiel, 2004), whereby exogenous genetic information is delivered to the cancer patient with the aim of effecting tumour ablation. The majority of cancer gene therapy and CBT approaches involve strategies either to suppress the function of activated oncogenes, to restore the expression of func- tional tumour suppressor genes, to potentiate the anti-tumour activity of the immune system, to down-regulate angiogenesis and metastasis, or to initiate tumour self-destruction (Seth, 2005). The latter strategy can be accomplished either via the intratumoural delivery and expression of so-called ‘‘toxic genes’’, which encode pro- teins which cause cell death directly (Agarwal et al., 2006; Johannes and Decaudin, 2005), or by the expression of so-called ‘‘suicide genes’’, which encode enzymes which are not toxic per se, but which catalyze the formation of highly toxic metab- olites following the application of a much less toxic prodrug. The use of suicide gene/prodrug combinations in this way is known as gene directed enzyme prodrug 6 D. Portsmouth et al. / Molecular Aspects of Medicine 28 (2007) 4–41 therapy (GDEPT). Although each of these strategies has its own set of advantages and drawbacks, detailed discussion of them all is beyond the scope of this review, which will focus solely on the enzyme/prodrug combinations currently being devel- oped for GDEPT. Similarly, although any GDEPT strategy can only be as good as the method used to deliver the therapeutic gene to its target, vector design has been covered in detail in several recent review articles (Dalba et al., 2005; Dong and Woraratanadharm, 2005; Kaneda and Tabata, 2006) and will not be discussed here. Suffice to say, potential side effects induced by any suicide gene will be minimised if its introduction and expression can be confined to target cells, and its efficacy will be maximised if it can be introduced to as many target cells as possible. GDEPT is synonymous with molecular chemotherapy, enzyme-activating pro- drug therapy and gene prodrug activation therapy (GPAT), terms which can all be interchangeably used to describe a process whereby the expression of exogenous genetic information generates a product capable of increasing the toxicity of an administered prodrug. GDEPT can either take the form of CBT, whereby cells genetically modified to express a suicide gene are placed in or near to the target tumour in the cancer patient, or of gene therapy, whereby the suicide gene is intro- duced to and expressed in the cancer cells themselves. GDEPT can also take the form of virus directed enzyme prodrug therapy (VDEPT), which refers to those strategies which make use of viral vectors for delivery of the suicide gene (Seth, 2005). None of these terms should be confused with antibody directed enzyme prodrug therapy (ADEPT), however, which refers only to the administration of enzyme-antibody conjugates (Sharma et al., 2005), does not involve the introduction of genetic infor- mation, and hence will not be discussed in this review. The potential advantages which GDEPT offers compared to classical chemo- therapy are multifold. Firstly, prodrugs can be used which have no intrinsic activ- ity in unmodified human cells. Secondly, concentrations of the toxic agent in the tumour can be much higher than in off-target organs since the prodrug is activated only in the target cells or in cells in close proximity to the target cells. Thirdly, not only the gene delivery vectors but also the suicide gene/prodrug combinations themselves can be designed to confer tumour specificity/selectivity on the treatment regime, e.g. by the use of tumour-specific regulatory elements to drive expression of the therapeutic gene (Saukkonen and Hemminki, 2004), or by the use of prodrugs which are preferentially active in hypoxic cells (Yakkundi et al., 2006), further reducing off-target toxicity. Fourthly, the use of suicide gene/prodrug combinations which allow the intercellular transport of either the gene product and/or the acti- vated prodrug generate a so-called ‘‘bystander effect’’, which allows the ablation of entire tumours even if not all of the target cells have been transduced with the sui- cide gene. In much the same way as the search for an ideal vector system is still ongoing, the ideal suicide gene/prodrug combination also remains to be discovered, and it seems increasingly likely that different approaches will be needed in different clinical set- tings. There is, however, a clear set of properties that the, as yet non-existent, ‘‘per- fect’’ suicide gene/prodrug combination should exhibit, including, for the suicide gene/gene product: D. Portsmouth et al. / Molecular Aspects of Medicine 28 (2007) 4–41 7 (i) to be either absent in the human genome, or expressed only at low levels in healthy organs, and be intrinsically non-toxic, (ii) to exhibit high catalytic activity upon expression in tumours even when the concentration of the prodrug is low (high KCAT, low Km), (iii) to be both necessary and sufficient for full activation of the prodrug without the necessity for further catalysis by endogenous enzymes, which could be mutated in some tumours, (iv) to be monomeric, allowing easier modification, and small, allowing the use of expression vectors in which the transgene choice is size-restricted, and, for the prodrug: (i) to have high affinity for the enzyme encoded by the suicide gene and low affin- ity for endogenous enzymes outside the tumour mass, (ii) to be able to penetrate into a solid tumour and be efficiently taken up by tumour cells, (iii) to exhibit no toxicity prior to activation, subsequent to which it should become highly toxic, (iv) that the toxic form(s) of the drug should be capable of intercellular diffusion to allow killing also of tumour cells via the bystander effect, (v) to have a half life long enough to maximise the bystander effect
Details
-
File Typepdf
-
Upload Time-
-
Content LanguagesEnglish
-
Upload UserAnonymous/Not logged-in
-
File Pages38 Page
-
File Size-