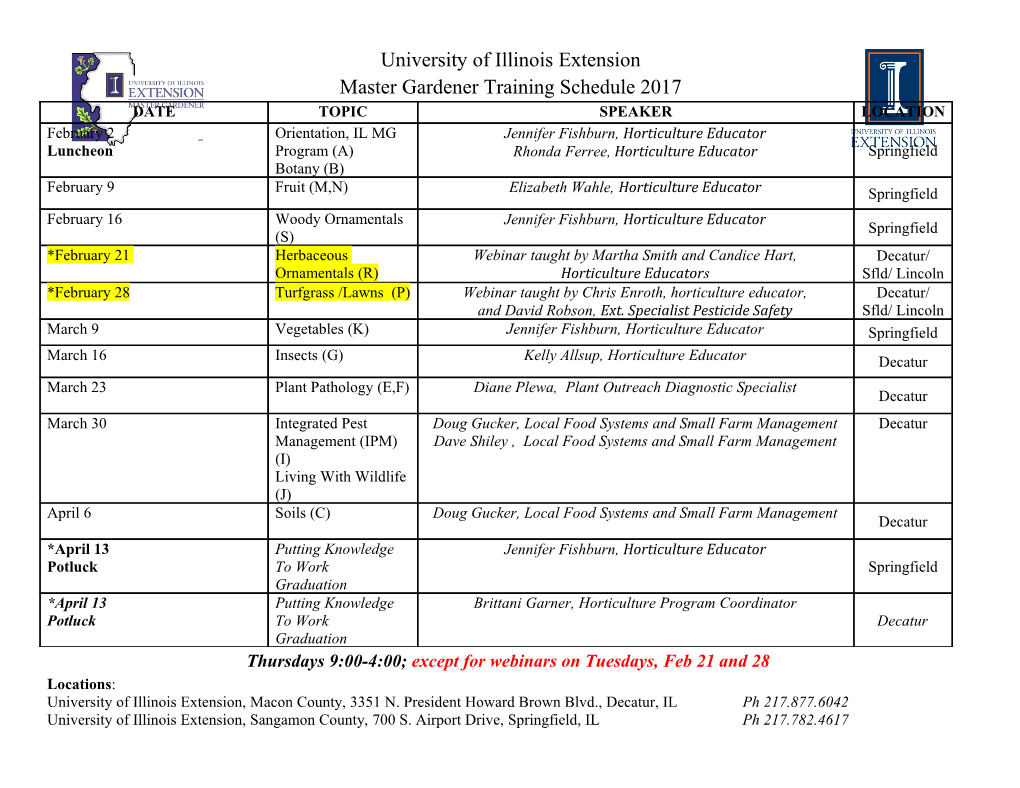
4 • Ecosystem and Plant Response e to Environmental Variables c O S y S T e m and Climate Change Biotic and Abiotic Interactions in Ecosystems a n d lants live in complex environments where many biotic and abiotic factors limit or promote productivity and carbon biosequestration. Temperature P and water extremes, nutrient and other resource availabilities, microbe- Pinduced diseases, and insect attacks can limit plant productivity. At the same l a n T time, this productivity intimately depends on plant interactions with certain beneficial microorganisms (as described in Chapter 3, Carbon Flows in Ecosys­ tems, p. 27) and access to appropriate temperature, water, and light regimes. r Furthermore, these biotic and abiotic factors can influence each other, giving rise e S P O n S e to complex environmental conditions to which plants must adapt. This complex­ ity is illustrated by millions of acres of bark-beetle outbreaks triggered by warm­ ing climates that in turn increase winter survival of bark-beetle populations. In the coming decades, such ecological complexities will pose increasing challenges in both agricultural and natural ecosystems (see Chapter 5, Ecosystem Dynam­ ics, p. 71, for a discussion on the impacts of climate change on the frequency and severity of such disturbances). Uncertainty about how abiotic and biotic factors interact at a mechanistic level limits a comprehensive understanding of plant and ecosystem productivity. This knowledge gap reduces our ability to interpret observations, make meaningful projections concerning disturbance and its impacts, and develop the strategies needed for intervening in an ecosystem’s response to abiotic and biotic inter­ actions. Thus, determining which abiotic and biotic factors most affect plant productivity, the mechanisms by which these factors act, and whether particular factors influence the quality of biomass accumulation (e.g., transient versus stable biomass) is essential for predicting ecosystem response. Moreover, such knowledge could reveal strategies to either enhance or diminish the extent to which particular interactions affect improved carbon biosequestration. Consequently, achieving increased plant productivity and carbon biosequestration requires studying and managing abiotic and biotic processes and interactions at multiple levels of organization—from molecular biology to whole-organism pheno­ types to ecological communities to global factors that influence Earth’s carbon cycle and climate change. Water Factors in Ecosystem Productivity Potential alterations in water availability arising from climate change will have significant implications for plant productivity. For example, climatic changes are expected to affect the overall rainfall quantity in many parts of the world, undoubtedly influencing plant growth. More subtle shifts in rainfall patterns throughout the year also might profoundly impact plant and plant-community growth patterns. Additionally, climate warming will alter soil water balance Carbon Cycling and Biosequestration Workshop U.S. Department of Energy Office of Science 59 4 • Ecosystem and Plant Response ­ Key Research Questions irrespective of changes in precipitation. The extent of such alterations will depend 1. What are the abiotic and on soil type. For example, soils unable to absorb and retain moisture may be biotic factors and interac­ affected more severely than others able to do so. Moreover, changes in soil water tions that determine nutrient content will have downstream effects on microbial communities and chemical availability? and nutrient mobility in soils. Likewise, climatic changes altering rainfall pH (i.e., acidification of rainwater) could have broad impacts on the chemical composi­ 2. How are the carbon, nutrient, tion, bioavailability of inorganic nutrients, and microbial communities in soil. and water cycles linked, and A comprehensive understanding of these complex, climate-induced changes is how do such linkages deter­ needed for accurate, predictive modeling of the effects of altered water availability mine ecosystem productivity, on plant productivity. carbon biosequestration, and response to climate change? Plant Traits and Strategies for Combatting Drought Plants possess a variety of strategies—some more successful than others—for dealing with water limitation. Such strategies fall into one of three general catego­ ries: (1) drought escape, reflecting plants’ ability to alter their life cycle to escape periods of water shortages; (2) drought avoidance, in which plants adjust inter­ nal processes to maintain their internal water supply; and (3) drought tolerance, which enables plants to continue to grow, though perhaps in an altered manner, despite reduced water (Bray 1997). Limited understanding of the mechanisms directing these strategies impedes our ability to optimize plant traits and produc­ tivity amid water deprivation. However, two traits—water use efficiency (WUE) and root systems—have been focuses in research to enhance plant productivity during drought. Defining Genes and Processes that Determine Water Use Efficiency Water is central to the distribution and productivity of plants in ecosystems and agriculture. Changing rainfall and temperature patterns give impetus to deter­ mining the molecular and developmental mechanisms that influence water use efficiency and plant productivity during drought. Experimental approaches in model plant species and crops have begun to identify causal influences on drought tolerance arising from various plant WUE strategies, including adaptations of traits for stomata, transpiration, root architecture, and other diverse physiologi­ cal mechanisms. The role of symbiotic fungi in water-deprivation adaptation also must be considered. Combined research approaches using systems biology, omics technologies, spectral analyses of water-stressed plants, and whole-plant pheno­ typic analyses of natural genetic variation offer great potential for understanding and manipulating drought tolerance in plants. Such an integrated understanding and subsequent optimization strategies would have important implications for plant productivity and carbon biosequestration. Transpiration and Nutrient Acquisition Many global climate change variables—including precipitation, temperature, length of growing season, humidity, and radiation intensity—likely will affect water availability and use by plants. The significant and direct impact on pri­ mary productivity from climate-induced shifts in plant water status is commonly recognized. Less widely known, however, is that altered soil water availability and transpiration will have important secondary effects on plant acquisition of soluble nutrients, especially nitrate-N, calcium, magnesium, sulfur, and silicon. U.S. Department of Energy Office of Science Carbon Cycling and Biosequestration Workshop 60 4 • Ecosystem and Plant Response ­ Regulation of Root-System Architecture for Water Acquisition Numerous studies have shown that elevated CO2 changes root architecture, or the spatial configuration of root systems. Architecture traits significantly correlate with a plant’s ability to survive under water stress. For instance, lateral-root density, a key trait in determining productivity, is linked to plant performance when water is limited. Denser lateral roots facilitate more water uptake, thus allowing plants to perform better during drought. Root systems and the mechanisms by which they increase water capture vary widely among drought-tolerant plants. In some plants, roots extending well below the surface obtain water deep into the soil profile. In others, shallow root systems allow rapid capture of rainwater before it is lost to evaporation. Some plants are highly plastic, having root systems that change in response to water availability or shift during development to adjust to seasonal fluctuations in soil water distribution. Moreover, hydrotropism, though poorly understood, is a process allowing roots to sense and grow toward water. These vari­ ous types of root systems are clearly important to plant productivity and survival during water stress. Thus a thorough understanding of the mechanisms regulating their development and the potential consequences of climate change on root archi­ tecture is critical. Also needed is greater insight into how root-system architecture and interactions with rhizobia change in response to shifts in water distribution. Root Architecture and Nutrient Acquisition The role of root architecture in mediating plant response to climate change will depend on ecosystem edaphic constraints—the limitations arising from specific soil conditions. Most terrestrial ecosystems have multiple such constraints, includ­ ing low availability of nitrogen, phosphorus, and often calcium, as well as exces­ sive levels of aluminum, manganese, or salinity. Although root-system response to elevated CO2 and nitrogen has received some research attention, few studies have investigated how shifts in root architecture affect other nutritional constraints. For example, architectural changes arising from elevated CO2 may have very different impacts when comparing plant acquisition of phosphorus and calcium, nutrients often limiting in forest soils. Phosphorus is diffusion-mobile, and calcium moves by mass water flow. Thus, architectural changes resulting in finer branching or root proliferation in topsoil may increase phosphorus acquisition, and those resulting in root extensions into deep areas with greater water availability might enhance
Details
-
File Typepdf
-
Upload Time-
-
Content LanguagesEnglish
-
Upload UserAnonymous/Not logged-in
-
File Pages12 Page
-
File Size-