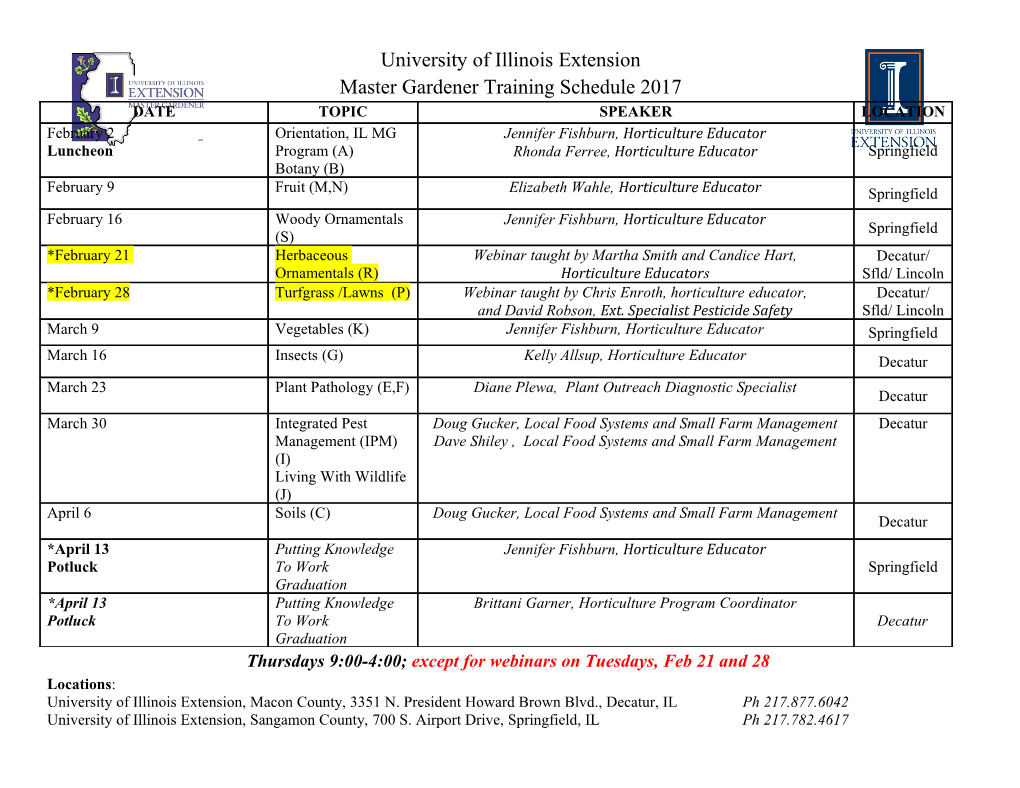
View metadata, citation and similar papers at core.ac.uk brought to you by CORE provided by Elsevier - Publisher Connector Chemistry & Biology Review Expanding the Genetic Code for Biological Studies Qian Wang,1 Angela R. Parrish,1 and Lei Wang1,* 1The Jack H. Skirball Center for Chemical Biology and Proteomics, The Salk Institute for Biological Studies, 10010 North Torrey Pines Road, La Jolla, CA 92037 *Correspondence: [email protected] DOI 10.1016/j.chembiol.2009.03.001 Using an orthogonal tRNA-synthetase pair, unnatural amino acids can be genetically encoded with high effi- ciency and fidelity, and over 40 unnatural amino acids have been site-specifically incorporated into proteins in Escherichia coli, yeast, or mammalian cells. Novel chemical or physical properties embodied in these amino acids enable new means for tailored manipulation of proteins. This review summarizes the method- ology and recent progress in expanding this technology to eukaryotic cells. Applications of genetically en- coded unnatural amino acids are highlighted with reports on labeling and modifying proteins, probing protein structure and function, identifying and regulating protein activity, and generating proteins with new proper- ties. Genetic incorporation of unnatural amino acids provides a powerful method for investigating a wide variety of biological processes both in vitro and in vivo. Introduction 1957; Minks et al., 2000; Link et al., 2003). For the site-specific The genetic code consists of 64 triplet codons specifying incorporation of unnatural amino acids, a suppressor tRNA is 20 canonical amino acids and 3 stop signals. This code is chemically acylated with an unnatural amino acid. When added preserved in all three kingdoms of life with few reassignments into cell extracts or Xenopus oocytes that support protein trans- (Knight et al., 2001). Although various amino acid derivatives lation, the acylated tRNA incorporates the attached unnatural have been identified in natural proteins, most of them are post- amino acid in response to a stop or extended codon (Noren translational modifications of the canonical amino acids (Uy et al., 1989; Bain et al., 1989; Nowak et al., 1995). All of these and Wold, 1977). Two additional amino acids, selenocysteine methods have proved to be extremely valuable, but each has (Bock et al., 1991) and pyrrolysine (Srinivasan et al., 2002), are limitations in the lack of site selectivity, the in vitro nature of the also found to be incorporated into proteins cotranslationally method, or the low incorporation efficiency (Wang and Schultz, and are thus regarded as natural expansions of the genetic 2004). code (Ambrogelly et al., 2006). With these amino acids and The ability to genetically encode unnatural amino acids in the with the help of posttranslational modifications and cofactors, same manner as the canonical amino acids would enable proteins are able to carry out various functions for sustaining life. specific changes to be precisely made in proteins directly Given the important roles of proteins in biology, it is desirable in vivo, thus providing novel tools for understanding biology in to be able to manipulate proteins at will for understanding protein molecular terms in the native settings. Here, we review the meth- structure-function relationships, investigating protein-involved odology for genetically incorporating unnatural amino acids into biological processes, and generating proteins and organisms proteins in live cells, and focus on recent advances in expanding with new properties. Therefore, tremendous efforts have been the method to eukaryotic cells. Representative applications are made regarding the incorporation of unnatural amino acids into discussed to illustrate the utility and scope of this technology proteins to introduce new functional groups apart from those in studying various biological questions. found in the canonical amino acids, through chemical or biosyn- Methodology thetic means (Wang and Schultz, 2004). Chemical methods use Similar to canonical amino acids, the genetic encoding of an chemistry to directly modify or prepare proteins. Early efforts unnatural amino acid requires a dedicated set of components focused on derivatizing reactive side chains of the protein, including a tRNA, a codon, and an aminoacyl-tRNA synthetase such as the thiol group of cysteine and the 3-amino group of (hereafter referred to as synthetase) (Figure 1)(Wang and lysine (Kaiser et al., 1985). The advance of stepwise solid-phase Schultz, 2002). This tRNA/codon/synthetase set, termed the peptide synthesis makes possible the complete synthesis of orthogonal set, must not crosstalk with endogenous tRNA/ peptides and small proteins (usually < 100 residues) containing codon/synthetase sets and must be functionally compatible any synthetically accessible unnatural amino acid (Kent, 1988). with other components of the translation apparatus. Specifically, The protein size limitation is subsequently overcome by semi- the orthogonal tRNA should not be recognized by any endoge- synthetic protein ligation methods, in which two or more peptide nous synthetase, and it should decode the orthogonal codon, fragments are chemically ligated to make the full-length protein which is not assigned to any canonical amino acid. The orthog- (Dawson et al., 1994; Muir, 2003). By contrast, biosynthetic onal synthetase should not charge any endogenous tRNA but methods harness the endogenous cellular machinery to translate the orthogonal tRNA, and it should charge the orthogonal the target protein, in which the unnatural amino acid is incorpo- tRNA with the unnatural amino acid only. The unnatural amino rated cotranslationally. Bacterial strains auxotrophic for a acid cannot be a substrate for any endogenous synthetase particular amino acid have been used to globally replace the and must be available in the cytoplasm of the host cell. Once amino acid with a close structural analog (Cohen and Cowie, expressed in cells, the orthogonal synthetase charges the Chemistry & Biology 16, March 27, 2009 ª2009 Elsevier Ltd All rights reserved 323 Chemistry & Biology Review Figure 1. A General Method for Genetically Encoding Unnatural Amino Acids in Live Cells orthogonal tRNA with the desired unnatural amino acid, and the (Ibba and Soll, 2000). As there is already a pool of tRNA/synthe- acylated tRNA incorporates the attached unnatural amino acid tase pairs inside cells, it is challenging to generate a new tRNA/ into proteins in response to the unique codon by utilizing the synthetase pair orthogonal to existing ones and functional in endogenous translational machinery. As all elements are genet- translation. A successful strategy is to import a tRNA/synthetase ically encodable, this method has the potential to be applied to pair from a species in a different kingdom of life, followed by fine almost all genetically tractable organisms. tuning with selections. This strategy is based on early reports Method Development in Escherichia coli showing that in vitro cross-species aminoacylation is often low Escherichia coli was initially chosen as the host organism to (Kwok and Wong, 1980). The first orthogonal tRNA/synthetase develop this general method for genetically encoding unnatural pair in E. coli generated from archaeal bacteria was derived amino acids in vivo, because its translational machinery had from the tRNATyr/tyrosyl-tRNA synthetase (TyrRS) from Metha- been extensively studied and genetic manipulation of E. coli is nococcus jannaschii (Wang et al., 2000). The anticodon of the efficient and relatively straightforward. A unique codon is tRNA is mutated to CUA for recognizing the amber codon Tyr Tyr required to specify the unnatural amino acid, and the amber UAG. This M. jannaschii tRNACUA (MjtRNACUA) and its cognate stop codon has been chosen for this purpose. There are three MjTyrRS function efficiently in translation in E. coli, but there is Tyr stop codons that do not code for amino acids but function as a small degree of aminoacylation of this MjtRNACUA by endoge- a signal for the termination of translation. By reassigning nous E. coli synthetases. To make a completely orthogonal tRNA a ‘‘blank’’ stop codon to an unnatural amino acid any overlap while maintaining its affinity for the cognate synthetase, a general and ambiguity with sense codons is avoided. The amber codon strategy has been developed to evolve orthogonal tRNAs in became the ‘‘blank’’ codon of choice in this approach because (i) E. coli (Figure 2A) (Wang and Schultz, 2001). A library of tRNA it is the least used among the three stop codons in E. coli, and mutants is prepared by randomizing specific nucleotides, and because (ii) amber suppressor tRNAs have been identified or the orthogonal tRNA is selected by using a combination of nega- engineered in E. coli to readthrough the amber codon with tive and positive selection. The negative selection removes canonical amino acids, and they do not significantly affect the nonorthogonal tRNAs from the library, and the subsequent posi- growth rates of E. coli cells (Normanly et al., 1990). Therefore, tive selection removes nonfunctional tRNAs but allows only specifying an unnatural amino acid with the amber codon was orthogonal tRNAs with high affinity for the cognate synthetase Tyr expected to minimize perturbations to the genetic code and to to pass. This approach was applied to the MjtRNACUA, and the Tyr the health of the host. mutRNACUA that is orthogonal in E. coli and functions Endogenous tRNAs and synthetases recognize their cognate
Details
-
File Typepdf
-
Upload Time-
-
Content LanguagesEnglish
-
Upload UserAnonymous/Not logged-in
-
File Pages14 Page
-
File Size-