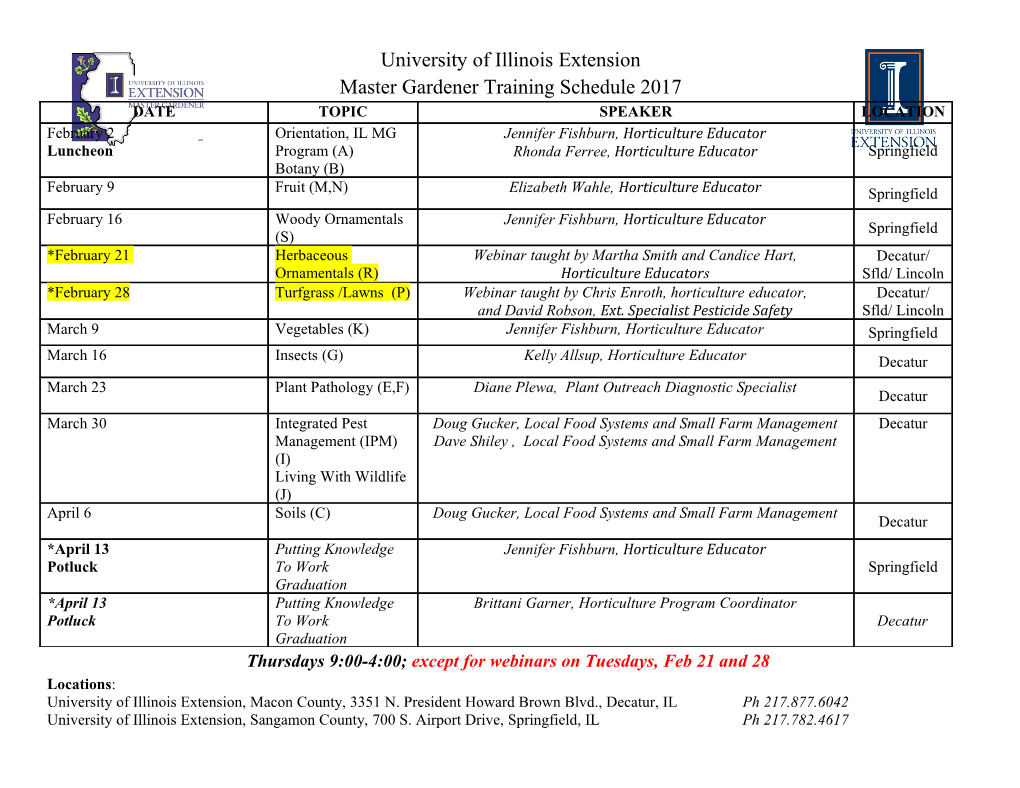
LETTERS PUBLISHED ONLINE: 5 JANUARY 2014 | DOI: 10.1038/NPHYS2848 Spin–layer locking effects in optical orientation of exciton spin in bilayer WSe2 Aaron M. Jones1, Hongyi Yu2, Jason S. Ross3, Philip Klement1,4, Nirmal J. Ghimire5,6, Jiaqiang Yan6,7, David G. Mandrus5,6,7, Wang Yao2 and Xiaodong Xu1,3* Coupling degrees of freedom of distinct nature plays a pseudospin up or down, respectively, which corresponds to electri- critical role in numerous physical phenomena1–10. The recent cal polarization. In a layered material with spin–valley coupling and emergence of layered materials11–13 provides a laboratory for AB stacking, such as bilayer TMDCs, both spin and valley are cou- studying the interplay between internal quantum degrees pled to layer pseudospin15. As shown in Fig. 1a, because the lower of freedom of electrons14,15. Here we report new coupling layer is a 180◦ in-plane rotation of the upper layer, the out-of-plane phenomena connecting real spin with layer pseudospins spin splitting has a sign that depends on both valley and layer pseu- in bilayer WSe2. In polarization-resolved photoluminescence dospins. Interlayer hopping thus has an energy cost equal to twice measurements, we observe large spin orientation of neutral the SOC strength λ. When 2λ is larger than the hopping amplitude and charged excitons by both circularly and linearly polarized t?, a carrier is localized in either the upper or lower layer depending excitation, with the trion spectrum splitting into a doublet on its valley and spin state. In other words, in a given valley, the at large vertical electrical field. These observations can be spin configuration is locked to the layer index. This is schematically explained as a locking of spin and layer pseudospin in illustrated in Fig. 1b,c. This spin–layer locking permits electrical a given valley15, where the doublet implies an electrically manipulation of spins through gate control of layer polarization, induced spin splitting. The observed distinctive behaviour of which may lead to new magnetoelectric effects and quantum logic15. the trion doublet under polarized excitation further provides Here, we report experimental signatures of coupling between spectroscopic evidence of interlayer and intralayer trion this layer pseudospin and the spin and valley degrees of freedom species, a promising step towards optical manipulation in van in bilayers of WSe2 (bi-WSe2). In contrast to MoS2 (ref. 19), the 16 der Waals heterostructures through interlayer excitons. high quality of WSe2, in addition to much larger SOC, provides Exploring the consequences of the interplay between distinct an excellent system for observing spin–layer locking. Although bi- quantum degrees of freedom has been an active theme in WSe2 is an indirect-bandgap semiconductor, the near degeneracy modern physics. A salient example is spin–orbit coupling (SOC), between indirect and direct transitions permits us to efficiently which is essential in renowned condensed matter phenomena monitor direct-gap photoluminescence from the K valleys12,13,27. such as the spin Hall effect1,2, topological insulators3,4 and Electrons in the conduction bands near the K valleys exhibit a Majorana fermions5,6; in cold atom physics in the search for new spin splitting 2λ ∼ 30–40 meV, which is two orders of magnitude 7 condensate structures ; and in technological applications such as larger than the interlayer hopping amplitude t? at the K points magnetoelectric coupling in multiferroics8 as well as optical and (see Supplementary Information 1). For holes, 2λ ∼ 450 meV and 9,10 electrical control of spins for spintronics . All of these phenomena 2λ=t? ∼ 7 (ref. 15). Large 2λ to t? ratios ensure that interlayer arise from the coupling of the motional degree of freedom of a hopping for both electrons and holes is suppressed, achieving a particle with its real spin. spin–layer locking effect. A pseudospin describes another discrete internal degree of We first identify the exciton states in bi-WSe2 through gate- freedom of electrons, and in most systems has an orbital origin and dependent photoluminescence measurements (see Methods)28,29. can therefore couple to the real spin by SOC as well. An excellent Figure 2a shows the photoluminescence intensity from direct-gap example is found in monolayer transition-metal dichalcogenides exciton emission as a function of backgate voltage (Vg) and (TMDCs), which have attracted a significant amount of interest photon energy. By comparing the gate-dependent patterns and recently12,13,17–21. The inversion symmetry breaking allows for an emission energies of monolayer (Supplementary Section 2)29 and 14 effective coupling between the real spin and valley pseudospin bilayer WSe2, we can identify the weak feature near 1.74 eV and o (the latter indexes the degenerate extrema of the electron energy Vg D 0 as neutral exciton (X ) emission, whereas the peak near − dispersion in momentum space). In the presence of mirror and 1.71 eV at positive or negative Vg corresponds to negative (X ) time-reversal symmetry, SOC can be manifested as an out-of-plane or positive (XC) trions, respectively. The photoluminescence peak spin splitting with a valley-dependent sign (Fig. 1a). near 1.63 eV arises from impurity-bound excitons. Figure 2c shows Bilayer two-dimensional materials (for example, bilayer photoluminescence spectra at three selected values of Vg. The peaks graphene22–26 and bilayer TMDCs; ref. 15) possess another distinct here coincide with the lowest energy absorption feature shown degree of freedom known as the layer pseudospin. An electronic in Fig. 2b, the gate-dependent differential reflectivity obtained by state localized to the upper or lower layer can be labelled with white-light reflection (Supplementary Section 3). 1Department of Physics, University of Washington, Seattle, Washington 98195, USA, 2Department of Physics and Center of Theoretical and Computational Physics, University of Hong Kong, Hong Kong, China, 3Department of Materials Science and Engineering, University of Washington, Seattle, Washington 98195, USA, 4Department of Physics, Justus-Liebig-University, Giessen 35392, Germany, 5Department of Physics and Astronomy, University of Tennessee, Knoxville, Tennessee 37996, USA, 6Materials Science and Technology Division, Oak Ridge National Laboratory, Oak Ridge, Tennessee 37831, USA, 7Department of Materials Science and Engineering, University of Tennessee, Knoxville, Tennessee 37996, USA. *e-mail: [email protected] 130 NATURE PHYSICS j VOL 10 j FEBRUARY 2014 j www.nature.com/naturephysics © 2014 Macmillan Publishers Limited. All rights reserved. NATURE PHYSICS DOI: 10.1038/NPHYS2848 LETTERS a Upper b K c Γ Upper layer Lower layer σ = 1 z |K,⇓ 〉 Lower σ ¬ σ + Γ ⇑ 〉 σ |K,⇑ z = ¬1 2λ τ ⇑ K( z = 1) τ ¬K( z = ¬1) ◦ Figure 1 j Coupled spin, valley and layer degrees of freedom in bilayer WSe2. a, AB stacking order in bilayer TMDCs corresponds to 180 rotation of the lattice between layers, leading to an effective layer pseudospin σz. Tz and 0 indicate the valley index and first Brillouin zone centre, respectively. b, Cartoon depicting excitation/emission processes in the K valley of bilayer WSe2. Spin configuration is indicated by * (") for holes (electrons). The same for the −K valley is obtained by time reversal. c, Depiction of spin-down (-up) hole states localized in the upper (lower) layer in the K valley. ac60 X¬ 60 V 40 18 15 Photoluminescence (counts s X¬ 20 12 Xo 0 6 ¬1 ) Gate (V) ) ¬20 ¬1 0 0 V 0 ¬40 18 X+ b ± 60 12 6 X¬ 40 0.2 Xo 20 0 Δ Photoluminescence (counts s ¬60 V R/R 18 0 Gate (V) X+ ¬20 12 0.0 ¬40 6 12 ¬60 0 1.601.65 1.70 1.75 1.601.65 1.70 1.75 Energy (eV) Energy (eV) Figure 2 j Photoluminescence and differential reflectivity versus gate. a, Photoluminescence intensity as a function of gate voltage and photon energy with labelled neutral exciton (Xo), and negative (X−) and positive (XC) trion emission. b, Corresponding differential reflectance showing relationship of X−=XC emission to the absorption feature. c, Photoluminescence spectra extracted at C60, 0 and −60 V, showing excitonic peaks X−,Xo and XC, respectively. Red lines in the 0 V spectrum show Lorentzian peak fits. Next we show that large and electrically tunable exciton spin view of ησ centred at 0 V, where an enhancement of an already large polarization can be generated in bi-WSe2 by optical pumping. ησ for increasing jVgj is observed. The doublet emerges for Vg above Figure 3a shows the polarization-resolved photoluminescence 60 V with differing degrees of exciton spin orientation for the two C C − C under σ excitation for σ (black curve) and σ (red curve) peaks. For simplicity, we plot just the σ branch of ησ , with the detection, at selected Vg. The degree of polarization is large for black and blue dots indicating the polarization of trion peaks I and Xo,XC and X− at all voltages, demonstrating the generation of II, respectively, obtained from the peak fittings (see Supplementary large exciton spin polarizations. Interestingly, for Vg above 60 V, a Section 4 for fitting parameters). Figure 3c shows the peak splitting − C C doublet structure emerges in the X spectrum for σ (co-polarized) as a function of Vg under σ -polarized excitation (black squares). detection, with an increasing separation between peaks at higher The gate-dependent photoluminescence in Fig. 2a shows the C − Vg. We label the peaks I and II as shown in the bottom right of crossover between X and X near Vg D 0, which demonstrates Fig. 3a. Note that the position of peak II coincides with the single that the sample is nearly intrinsic, without substantial external peak present for σ − (cross-polarized) detection. The doublet can be doping from substrate effects or impurities. The inset in Fig.
Details
-
File Typepdf
-
Upload Time-
-
Content LanguagesEnglish
-
Upload UserAnonymous/Not logged-in
-
File Pages5 Page
-
File Size-