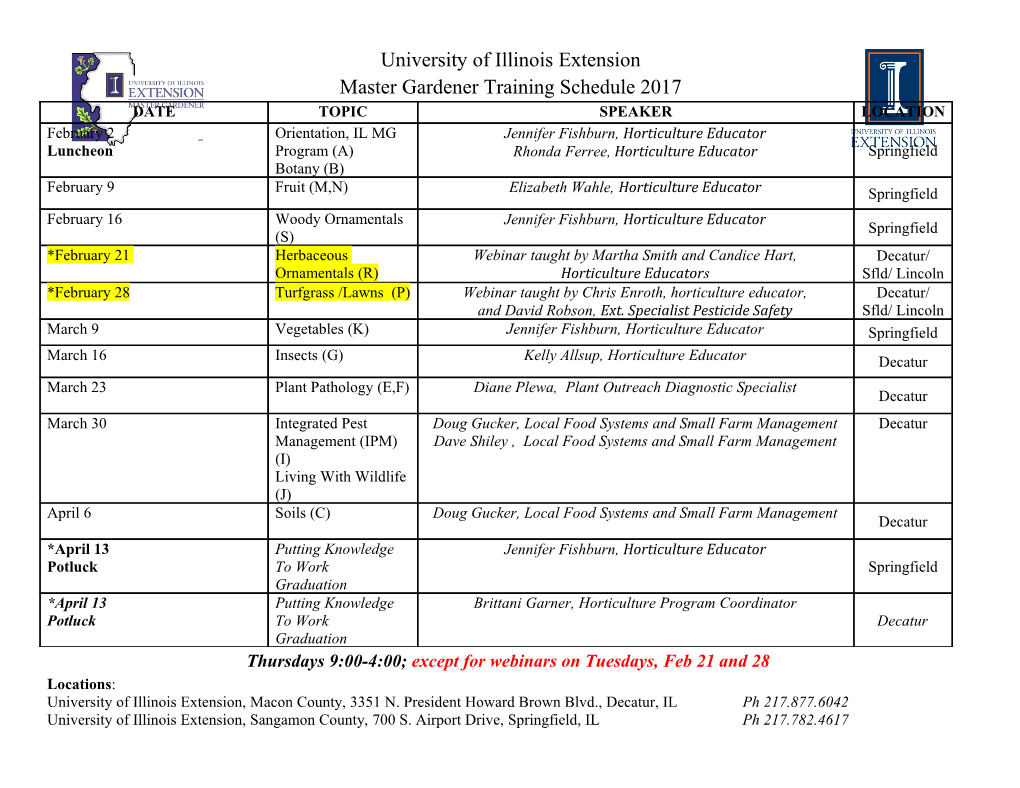
bioRxiv preprint doi: https://doi.org/10.1101/764225; this version posted September 10, 2019. The copyright holder for this preprint (which was not certified by peer review) is the author/funder, who has granted bioRxiv a license to display the preprint in perpetuity. It is made available under aCC-BY-NC-ND 4.0 International license. 1 Animal biosynthesis of complex polyketides in a photosynthetic partnership 2 Joshua P. Torres1, Zhenjian Lin1, Jaclyn M. Winter1, Patrick J. Krug2, and Eric W. Schmidt1* 3 1Department of Medicinal Chemistry, University of Utah, Salt Lake City, UT 84112 USA 4 2Department of Biological Sciences, California State University, Los Angeles, CA 90032 USA 5 *Correspondence to: [email protected] 6 7 ABSTRACT 8 Animals are rich sources of complex polyketides, including pharmaceuticals, cosmetics, and 9 other products. Most polyketides are associated with microbial or plant metabolism1. For this 10 reason, symbiotic bacteria or dietary organisms are often the true producers of compounds found 11 in animals2,3. Although increasing evidence suggests that animals themselves make some 12 compounds4-7, the origin of most polyketides in animals remains unknown. This problem makes 13 it difficult to supply useful animal compounds as drugs and severely constrains our 14 understanding of chemical diversity and the scope of biosynthesis in nature. Here, we 15 demonstrate that animals produce microbe-like complex polyketides. We report a previously 16 undocumented but widespread branch of fatty acid synthase- (FAS)-like proteins that have been 17 retooled by evolution to synthesize complex products. One FAS-like protein uses only 18 methylmalonyl-CoA as a substrate, otherwise unknown in animal lipid metabolism, and is 19 involved in an intricate partnership between a sea slug and captured chloroplasts. The enzyme’s 20 complex, methylated polyketide product results from a metabolic interplay between algal 21 chloroplasts and animal host cells, and also likely facilitates the survival of both symbiotic 22 partners, acting as a photoprotectant for plastids and an antioxidant for the slug8-12. Thus, we find bioRxiv preprint doi: https://doi.org/10.1101/764225; this version posted September 10, 2019. The copyright holder for this preprint (which was not certified by peer review) is the author/funder, who has granted bioRxiv a license to display the preprint in perpetuity. It is made available under aCC-BY-NC-ND 4.0 International license. 23 that animals can unexpectedly synthesize a large and medically useful class of structurally 24 complex polyketides previously ascribed solely to microbes, and can use them to promote 25 symbiotic organelle maintenance. Because this represents an otherwise uncharacterized branch 26 of polyketide and fatty acid metabolism, we anticipate a large diversity of animal polyketide 27 products and enzymes awaiting discovery. 28 29 MAIN 30 Complex, branched polyketides, are canonically ascribed to microbial metabolism, yet they are 31 commonly found in animals13,14. The sacoglossan polypropionate pyrones are among the most 32 structurally complex polyketides isolated from animals (Fig. 1). Sacoglossans consume algae, 33 and some species maintain the stolen algal chloroplasts (kleptoplasts) for weeks or in exceptional 34 cases, for several months15. Feeding studies showed that fixed carbon obtained from de novo 35 photosynthesis is efficiently incorporated into polypropionates via chloroplasts16. Labeled 36 propionate is also incorporated17-20, suggesting that the mollusk compounds are made via the 37 methylmalonate pathway, which has historically been associated with bacterial metabolism24. 38 The polypropionates react via oxidative and photocyclization pathways that are likely non- 39 enzymatic to form the mature natural products8-10,16. It is thought that these reactions protect the 40 slugs from damage associated with photosynthesis, and that they may thus be necessary for a 41 photosynthetic lifestyle8-12. Thus, the polypropionates are known to originate in the mollusks and 42 to be central to mollusk biology, yet their biochemical sources were unknown. 43 bioRxiv preprint doi: https://doi.org/10.1101/764225; this version posted September 10, 2019. The copyright holder for this preprint (which was not certified by peer review) is the author/funder, who has granted bioRxiv a license to display the preprint in perpetuity. It is made available under aCC-BY-NC-ND 4.0 International license. 44 We took an unbiased approach to define the molecular source of polypropionates. To test the 45 bacterial origin hypothesis, we sequenced the metagenomes of polypropionate-containing 46 sacoglossans Plakobranchus cf. ocellatus “aff. sp. 1”21 and Elysia diomedea. We were unable to 47 identify good candidate polypropionate synthases from the bacterial metagenomes, suggesting a 48 possible origin in the animals or chloroplasts. We took advantage of recently released genomes 49 and transcriptomes of three sacoglossans11,22-24, Elysia chlorotica, E. timida, and E. cornigera, to 50 test this hypothesis. E. chlorotica contains polypropionates ent-9,10-deoxytridachione and 51 elysione25, while E. timida contains ent-9,10-deoxytridachione and several relatives (Fig. 1, 52 Extended Data Table 1)26. Both species obtain fixed carbon from chloroplasts that they maintain 53 for one (E. timida) or several (E. chlorotica) months. In contrast, no polypropionates have been 54 reported from E. cornigera, which only retains chloroplasts for a few days27. 55 Fig. 1: Biogenesis of sacoglossan polypropionates. 56 bioRxiv preprint doi: https://doi.org/10.1101/764225; this version posted September 10, 2019. The copyright holder for this preprint (which was not certified by peer review) is the author/funder, who has granted bioRxiv a license to display the preprint in perpetuity. It is made available under aCC-BY-NC-ND 4.0 International license. 57 a Previous feeding studies demonstrated that kleptoplasts in the sacoglossans fix carbon that is 58 used to make polypropionates. Propionate is also a precursor, presumably via methylmalonate. 59 A further series of hypothetical steps, including synthesis by a PKS, methylation, and 60 photochemical and oxidation reactions would lead to b the known natural polypropionates of 61 diverse structures. These are found in c sacoglossan species investigated in this work, including 62 E. chlorotica and E. timida, but not E. cornigera. 63 64 Based upon recent reports of animals that make polyene fatty acids5,6, we suspected that the 65 animals might encode modified fatty acid synthase (FAS) or PKS enzymes. Examining the 66 available E. chlorotica transcriptomes, we found a number of fragmented transcripts encoding 67 multiple type I FAS enzymes. Reassembly of those transcripts from raw read data revealed four 68 different FAS-like enzymes (Extended Data Table 2). Two of these were the cytoplasmic and 69 mitochondrial FAS enzymes of primary metabolism. The remaining two (which we named 70 EcPKS1 and EcPKS2) formed their own distinct group in a global PKS/FAS tree that included 71 bacterial, fungal, and animal PKS variants (Fig. 2A, Extended Data Fig. 1). EcPKS1, EcPKS2, 72 and cytoplasmic FAS were encoded in the animal genomes, and not in chloroplasts (Fig. 2C, 73 Extended Data Fig. 2). EcPKS1 homologs were only found in E. chlorotica and E. timida, and 74 not in E. cornigera, while the other genes were found in all three. This observation was 75 consistent with EcPKS1 being the putative polypropionate synthase. 76 77 Like other type I FAS and PKS enzymes, EcPKS1 and EcPKS2 contain multiple domains 78 responsible for product formation (Fig. 2B). Enoylreductase (ER) domains reduce double bonds bioRxiv preprint doi: https://doi.org/10.1101/764225; this version posted September 10, 2019. The copyright holder for this preprint (which was not certified by peer review) is the author/funder, who has granted bioRxiv a license to display the preprint in perpetuity. It is made available under aCC-BY-NC-ND 4.0 International license. 79 in the nascent polyketide chain. Because EcPKS1 and EcPKS2 lack functional ER domains, theyey 80 likely both synthesize polyenes (Fig. 2D)5,28. The acyltransferase (AT) domain is responsible forr 81 substrate selection, and therefore would be responsible for choosing malonyl-CoA (straight- 82 chain lipids) or methylmalonyl-CoA (methyl-branched lipids)29. In bacteria, amino acid residuess 83 responsible for AT selectivity are well understood30. Since no sequenced animal FASs/PKSs 84 prefer methylmalonyl-CoA, there is no model for AT selectivity in animals. Gratifyingly, 85 EcPKS1, EcPKS2, and several relatives, contained a conserved GHSXGE sequence motif found 86 in bacterial ATs that is known to be important in substrate selection. In bacteria, when X is 87 methionine, the substrate is methylmalonyl-CoA. In EcPKS1, a GHSMGE sequence was 88 observed, whereas that of EcPKS2 was GHSLGE (Fig. 2E). This implied that EcPKS1 might usese 89 methylmalonyl-CoA, while EcPKS2 should use malonyl-CoA. Thus, EcPKS1 had all of the 90 sequence and expression features consistent with the polypropionate synthase. 91 Fig. 2: Sacoglossan PKSs, a new class of FAS-like PKS enyzmes. 92 bioRxiv preprint doi: https://doi.org/10.1101/764225; this version posted September 10, 2019. The copyright holder for this preprint (which was not certified by peer review) is the author/funder, who has granted bioRxiv a license to display the preprint in perpetuity. It is made available under aCC-BY-NC-ND 4.0 International license. 93 a Sacoglossan PKSs represent a group separate from the known FAS and PKS proteins from 94 bacteria, fungi and animals. Numbers indicate consensus support in percent. Outgroup DpsA is 95 a type II PKS sequence from bacteria, while the remainder are type I enzymes. b The domain 96 architecture of the sacoglossan PKSs, EcPKS1 and EcPKS2 includes ketosynthase (KS), 97 acyltransferase (AT), methyltransferase (MT), dehydratase (DH), enoylreductase (ER), 98 ketoreductase (KR) and acyl carrier protein (ACP). This domain architecture is identical to the 99 sacoglossan FAS, EcFAS except for the absence of a C-terminal thioesterase domain.
Details
-
File Typepdf
-
Upload Time-
-
Content LanguagesEnglish
-
Upload UserAnonymous/Not logged-in
-
File Pages29 Page
-
File Size-