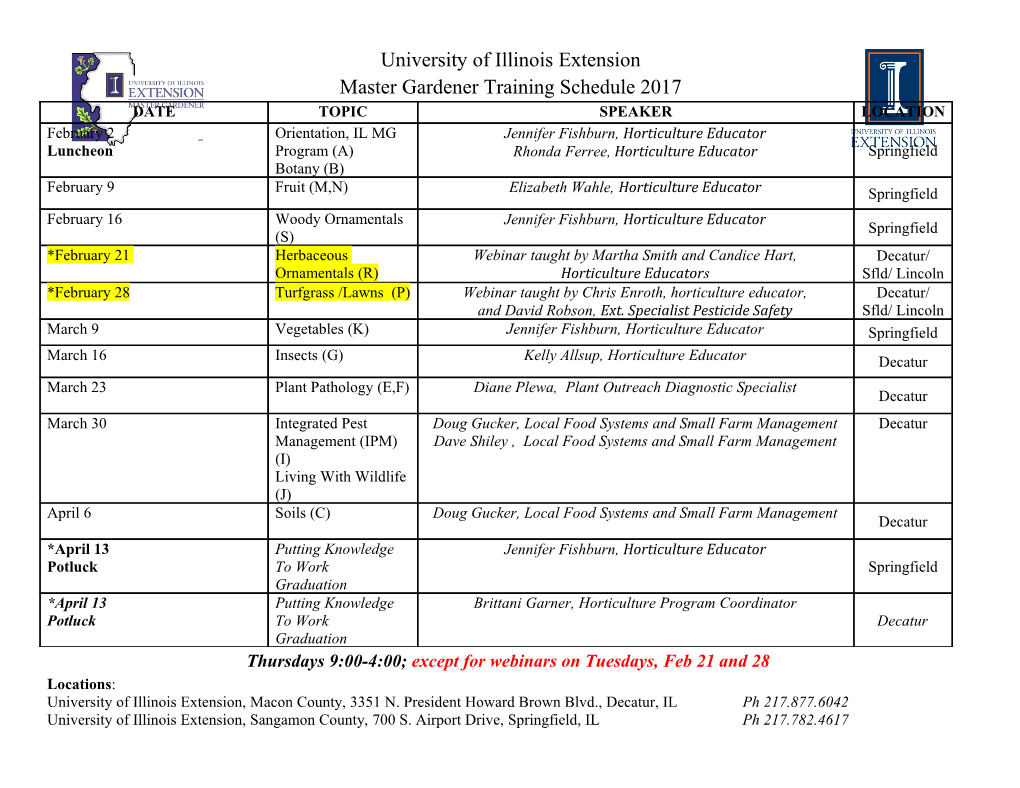
EPJ manuscript No. (will be inserted by the editor) arXiv:1508.03260 13 Aug 2015 and PREPRINT CERN-PH-TH-2015-194 Melting Hadrons, Boiling Quarks Johann Rafelski12 1 CERN-PH/TH, 1211 Geneva 23, Switzerland 2 Department of Physics, The University of Arizona Tucson, Arizona, 85721, USA Submitted: August 11, 2015 / Print date: September 17, 2015 Abstract. In the context of the Hagedorn temperature half-centenary I describe our understanding of the hot phases of hadronic matter both below and above the Hagedorn temperature. The first part of the review addresses many frequently posed questions about properties of hadronic matter in different phases, phase transition and the exploration of quark-gluon plasma (QGP). The historical context of the discovery of QGP is shown and the role of strangeness and strange antibaryon signature of QGP illustrated. In the second part I discuss the corresponding theoretical ideas and show how experimental results can be used to describe the properties of QGP at hadronization. The material of this review is complemented by two early and unpublished reports containing the prediction of the different forms of hadron matter, and of the formation of QGP in relativistic heavy ion collisions, including the discussion of strangeness, and in particular strange antibaryon signature of QGP. PACS. 24.10.Pa Thermal and statistical models { 25.75.-q Relativistic heavy-ion collisions { 21.65.Qr Quark matter { 12.38.Mh Quark-gluon plasma 1 Introduction A report on `Melting Hadrons, Boiling Quarks and TH ' relates strongly to quantum chromodynamics (QCD), the The year 1964/65 saw the rise of several new ideas which theory of quarks and gluons, the building blocks of had- in the following 50 years shaped the discoveries in funda- rons, and its lattice numerical solutions; QCD is the quan- mental subatomic physics: tum (Q) theory of color-charged (C) quark and gluon dy- 1. The Hagedorn temperature TH ; later recognized as the namics (D); for numerical study the space-time continuum melting point of hadrons into is discretized on a `lattice'. 2. Quarks as building blocks of hadrons; and, Telling the story of how we learned that strong inter- 3. The Higgs particle and field escape from the Goldstone actions are a gauge theory involving two types of parti- theorem, allowing the understanding of weak interac- cles, quarks and gluons, and the working of the lattice tions, the source of inertial mass of the elementary par- numerical method would entirely change the contents of ticles. this article, and be beyond the expertise of the author. I The topic in this paper is Hagedorn temperature TH recommend instead the book by Weinberg [8], which also and the strong interaction phenomena near to TH . I present shows the historical path to QCD. The best sources of the an overview of 50 years of effort with emphasis on: QCD relation to the topic of this article are: (a) the book a) Hot nuclear and hadronic matter; by Kohsuke Yagi and Tetsuo Hatsuda [9] as well as, (b) arXiv:1508.03260v2 [nucl-th] 16 Sep 2015 b) Critical behavior near TH ; the now 15 year old monograph by Letessier and the au- c) Quark-gluon plasma (QGP); thor [6]. We often refer to lattice-QCD method to present d) Relativistic heavy ion (RHI) collisions1; QCD properties of interest in this article. There are books e) The hadronization process of QGP; and many reviews on lattice implementation of gauge the- f) Abundant production of strangeness flavor. ories of interacting fields, also specific to hot-lattice-QCD This presentation connects and extends a recent retro- method. At the time of writing I do not have a favorite to spective work, Ref. [1]: Melting Hadrons, Boiling Quarks; recommend. From Hagedorn temperature to ultra-relativistic heavy-ion collisions at CERN; with a tribute to Rolf Hagedorn. This Immediately in the following Subsection 1.1 the fa- report complements prior summaries of our work: 1986 [2], mous Why? is addressed. After that I turn to answering 1991 [3],1996 [4], 2000 [5], 2002 [6], 2008 [7]. the How? question in Subsection 1.2, and include a few reminiscences about the accelerator race in Subsection 1.3. 1 We refer to atomic nuclei which are heavier than the α- I close this Introduction with Subsection 1.4 where the or- particle as `heavy ions'. ganization and contents of this review will be explained. 2 Johann Rafelski: Melting Hadrons, Boiling Quarks 1.1 What are the conceptual challenges of the into mass of matter. One can wonder aloud if this sheds QGP/RHI collisions research program? some light on the reverse process: Is it possible to convert matter into energy in the laboratory? Our conviction that we achieved in laboratory experi- The last two points show the potential of `applications' ments the conditions required for melting (we can also of QGP physics to change both our understanding of, and say, dissolution) of hadrons into a soup of boiling quarks our place in the world. For the present we keep these ques- and gluons became firmer in the past 15-20 years. Now tions in mind. This review will address all the other chal- we can ask, what are the `applications' of the quark-gluon lenges listed under points 1), 2), and 3) above; however, plasma physics? Here is a short wish list: see also thoughts along comparable foundational lines pre- 1) Nucleons dominate the mass of matter by a factor 1000. sented in Subsections 7.3 and 7.4. The mass of the three `elementary' quarks found in nu- cleons is about 50 times smaller than the nucleon mass. Whatever compresses and keeps the quarks within the 1.2 From melting hadrons to boiling quarks nucleon volume is thus the source of nearly all of mass of matter. This clarifies that the Higgs field provides the With the hindsight of 50 years I believe that Hagedorn's mass scale to all particles that we view today as elemen- effort to interpret particle multiplicity data has led to the tary. Therefore only a small %-sized fraction of the mass of recognition of the opportunity to study quark deconfine- matter originates directly in the Higgs field; see Section 7.1 ment at high temperature. This is the topic of the book [1] for further discussion. The question: What is mass? can be Melting Hadrons, Boiling Quarks; From Hagedorn temper- studied by melting hadrons into quarks in RHI collisions. ature to ultra-relativistic heavy-ion collisions at CERN; with a tribute to Rolf Hagedorn published at Springer 2) Quarks are kept inside hadrons by the `vacuum' prop- Open, i.e. available for free on-line. This article should erties which abhor the color charge of quarks. This expla- be seen as a companion addressing more recent develop- nation of 1) means that there must be at least two dif- ments, and setting a contemporary context for this book. ferent forms of the modern æther that we call `vacuum': How did we get here? There were two critical mile- the world around us, and the holes in it that are called stones: hadrons. The question: Can we form arbitrarily big holes filled with almost free quarks and gluons? was and remains I) The first milestone occurred in 1964{1965, when Hage- the existential issue for laboratory study of hot matter dorn, working to resolve discrepancies of the statistical made of quarks and gluons, the QGP. Aficionados of the particle production model with the pp reaction data, pro- lattice-QCD should take note that the presentation of two duced his \distinguishable particles" insight. Due to a phases of matter in numerical simulations does not answer twist of history, the initial research work was archived this question as the lattice method studies the entire Uni- without publication and has only become available to a verse, showing hadron properties at low temperature, and wider public recently; that is, 50 years later, see Chapter QGP properties at high temperature. 19 in [1] and Ref.[10]. Hagedorn went on to interpret the observation he made. Within a few months, in Fall 1964, 3) We all agree that QGP was the primordial Big-Bang he created the Statistical Bootstrap Model (SBM) [11], stuff that filled the Universe before `normal' matter formed. showing how the large diversity of strongly interacting Thus any laboratory exploration of the QGP properties particles could arise; Steven Frautschi [12] coined in 1971 solidifies our models of the Big Bang and allows us to ask the name `Statistical Bootstrap Model'. these questions: What are the properties of the primordial matter content of the Universe? and How does `normal' II) The second milestone occurred in the late 70s and early matter formation in early Universe work? 80s when we spearheaded the development of an experi- mental program to study `melted' hadrons and the `boil- 4) What is flavor? In elementary particle collisions, we ing' quark-gluon plasma phase of matter. The intense the- deal with a few, and in most cases only one, pair of newly oretical and experimental work on the thermal properties created 2nd, or 3rd flavor family of particles at a time. of strongly interacting matter, and the confirmation of a A new situation arises in the QGP formed in relativis- new quark-gluon plasma paradigm started in 1977 when tic heavy ion collisions. QGP includes a large number of the SBM mutated to become a model for melting nuclear particles from the second family: the strange quarks and matter. This development motivated the experimental ex- also, the yet heavier charmed quarks; and from the third ploration in the collisions of heavy nuclei at relativistic en- family at the LHC we expect an appreciable abundance ergies of the phases of matter in conditions close to those of bottom quarks.
Details
-
File Typepdf
-
Upload Time-
-
Content LanguagesEnglish
-
Upload UserAnonymous/Not logged-in
-
File Pages84 Page
-
File Size-