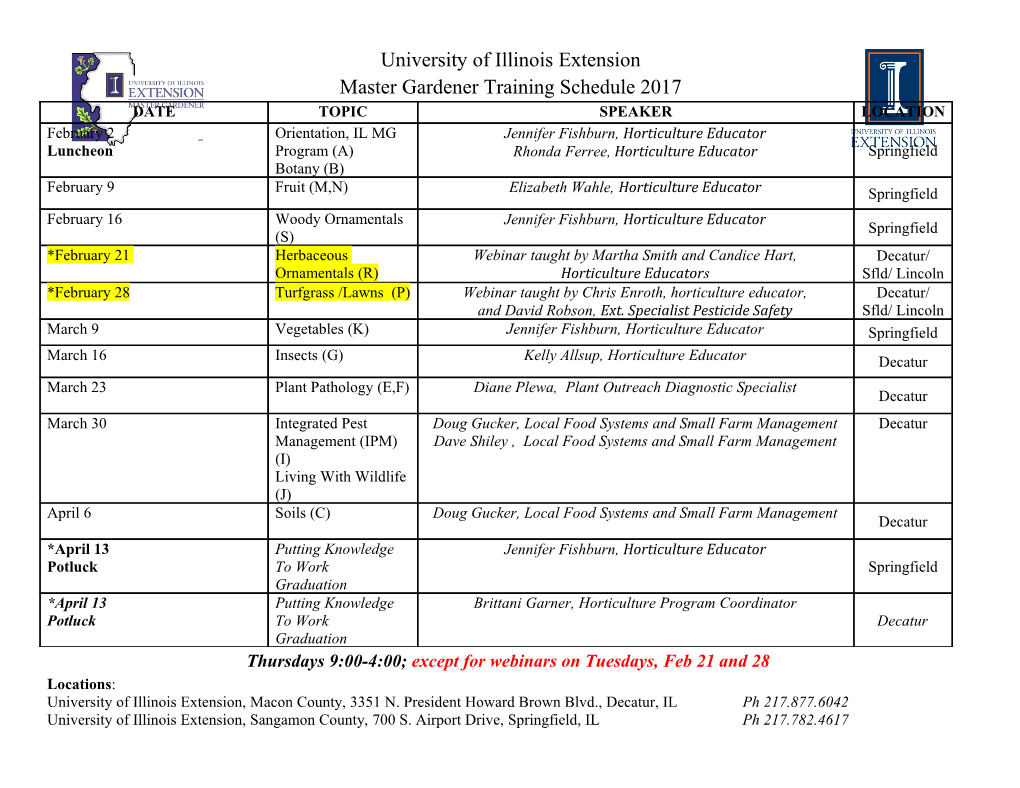
PHYSICAL REVIEW B 81, 205407 ͑2010͒ Bulk Fermi surface coexistence with Dirac surface state in Bi2Se3: A comparison of photoemission and Shubnikov–de Haas measurements James G. Analytis,1,2 Jiun-Haw Chu,1,2 Yulin Chen,1,2 Felipe Corredor,1,2 Ross D. McDonald,3 Z. X. Shen,1,2 and Ian R. Fisher1,2 1Stanford Institute for Materials and Energy Sciences, SLAC National Accelerator Laboratory, 2575 Sand Hill Road, Menlo Park, California 94025, USA 2Geballe Laboratory for Advanced Materials and Department of Applied Physics, Stanford University, Stanford, California 94305, USA 3Los Alamos National Laboratory, Los Alamos, New Mexico 87545, USA ͑Received 2 February 2010; published 5 May 2010͒ Shubnikov-de Haas ͑SdH͒ oscillations and angle-resolved photoemission spectroscopy ͑ARPES͒ are used to probe the Fermi surface of single crystals of Bi2Se3. We find that SdH and ARPES probes quantitatively agree on measurements of the effective mass and bulk band dispersion. In high carrier density samples, the two probes also agree in the exact position of the Fermi level EF, but for lower carrier density samples discrepan- cies emerge in the position of EF. In particular, SdH reveals a bulk three-dimensional Fermi surface for samples with carrier densities as low as 1017 cm−3. We suggest a simple mechanism to explain these differ- ences and discuss consequences for existing and future transport studies of topological insulators. DOI: 10.1103/PhysRevB.81.205407 PACS number͑s͒: 72.20.My, 03.65.Vf, 71.70.Di, 73.25.ϩi Recently, a new state of matter, known as a topological high level of consistency across all samples measured from insulator, has been predicted to exist in a number of materi- the same batch. While ARPES places EF near the SdH level 1,2 als: Bi1−xSbx,Bi2Se3,Bi2Te3, and Sb2Te3. This state of for some samples, there are others from the same batch matter is characterized by a full band gap in the bulk of the whose EF is found to reside into the bulk gap. We discuss material, but with a gapless, dissipationless surface state. The possible explanations for these discrepancies and the impli- surface state is comprised of counter-propagating spin states, cations for transport studies of surface Dirac fermions in which create a dispersion of a single, massless Dirac cone samples near a metal-insulator transition. that is protected by time-reversal symmetry. The experimen- The material Bi2Se3 can be grown without the introduc- tal realization of this state could mean significant advances in tion of foreign dopants as either n or p type12,13 though is spintronic devices, quantum computation, and much more more commonly found as the former because the dominant besides. defects tend to be Se vacancies. Quantum oscillatory phe- As a result there has been great excitement in the last year nomena, which provides evidence of bulk metallic behavior after the discoveries of various angle-resolved photoemission has been reported by Köhler12 on low carrier density samples spectroscopy ͑ARPES͒ experiments3–5 and more recently and more recently by Kulbachinskii14 on high carrier density from scanning-tunneling measurements6–8 that such a state samples. Below a carrier density of 7ϫ1018 cm−3, the band appears to exist in nature. Amidst this flurry of recent results, structure is well approximated by a single parabolic band, it is easy to forget that these same materials have been the making the interpretation of transport measurements subject of careful and thorough research for much of the 20th transparent.14 Two n-type samples with carrier densities dif- century. However, common to all the unambiguous measure- fering by 2 orders of magnitude are shown in Fig. 1. For the ments of the Dirac cone is the use of surface-sensitive low carrier density samples an upturn in the resistivity is probes. Only recently have transport measurements emerged seen, which levels off at sufficiently low temperature. This specifically investigating the surface state ͑Refs. 9–11͒, all of behavior has been attributed to the presence of an impurity which note the dominance of the bulk conductivity. It is thus band whose thermally activated conductivity is comparable of great interest to perform a coordinated study of these ma- to the band conductivity until carriers freeze out at around 30 terials using both bulk transport experiments and surface K.13,14 This behavior is not apparent in the higher carrier sensitive ARPES experiments. Here we report results of density materials, where the band conductivity always domi- these investigations. The transport experiments reveal quan- nates. Furthermore, the mobility increases as the carrier den- tum oscillations that indicate a bulk Fermi surface volume sity decreases, consistent with previous measurements.14 The that monotonically changes with carrier density. For carrier carrier densities of our highest mobility samples are around densities in the range ϳ1019 cm−3, the transport extracted an order of magnitude smaller than those of reported topo- ͑ ϳ band structure is in quantitative agreement with the bulk logical insulators including Sn-doped Bi2Te3 ne 1.7 ϫ 18 −3͒͑ ͒ ͑ ϳ band structure determined by ARPES which also observes 10 cm Ref. 4 or Ca-doped Bi2Se3 ne 5 the Dirac dispersion of the surface state. The quantitative ϫ1018 cm−3͒͑Ref. 5͒ and as a result may be better candi- agreement between ARPES and SdH provides additional dates in which to observe the transport properties dominated support for the existence of novel band structure in these by the topological surface state. materials. For lower carrier density samples down to Single crystals of Bi2Se3 have been grown by slow cool- 1017 cm−3 we observe SdH oscillations which unambigu- ing a binary melt. Elemental Bi and Se were mixed in alu- ͑ ously pin the Fermi level in the bulk conduction band, with a mina crucibles in a molar ratio of 35:65 for batch S1 ne 1098-0121/2010/81͑20͒/205407͑5͒ 205407-1 ©2010 The American Physical Society ANALYTIS et al. PHYSICAL REVIEW B 81, 205407 ͑2010͒ ͑ ͒͑͒ FIG. 1. Color online a Temperature dependence of two typical samples of Bi2Se3 with carrier densities differing by 2 orders of ͑ ͒ ͑ ͒ ͑ ͒ magnitude. b Shows the carrier density ne, c the resistivity 0 at T=2 K, and d the mobility for samples of different thicknesses. Each sample was a cleave from a parent sample, so that the surface area of each sample was kept constant. ϫ 17͒ ͑ ϫ 17͒ =5 10 , 34:66 for batch S2 ne =3 10 , 34:66 for tracted from SdH is in good quantitative agreement with that ͑ ϫ 17͒ ͑ batch S3 ne =2.3 10 , and 40:60 for batch S4 ne =2.3 measured by ARPES. ϫ1019͒. The mixtures were sealed in quartz ampules and In Fig. 3 we illustrate angle-dependent SdH data ͑a͒ taken raised to 750 °C and cooled slowly to 550 °C, then an- at 1.8 K, on a sample from batch S1 with a lower carrier nealed for an extended period. Crystals can be cleaved very density of 1017 cm−3. The SdH signal reveals a pocket that is easily perpendicular to the ͑001͒ axis. Measurements of the approximately an ellipsoid elongated about the c3 axis, con- resistivity and Hall effect were measured in a 14 T PPMS sistent with measurements by Köhler from the 1970s ͑Ref. using a standard four-probe contact configuration and Hall 12͒ on samples with similar carrier densities. For a two- measurements were performed using a six-probe configura- dimensional pocket expected from the surface state, quantum tion. For the latter, only data which were linear in the low- oscillations should vary as 1/cos , where is the angle field limit were used to avoid mixing with longitudinal com- between the c3 axis and the field direction, so the present ponents. In addition to this precaution, signal from positive observations must originate from a three-dimensional ͑3D͒ and negative field sweeps was subtracted to extract the odd Fermi surface existing in the bulk. It has been shown by ͑Hall͒ components of the signal, after which the carrier den- sity is extracted in the usual way. ARPES measurements were performed at beam line 10.0.1 of the Advanced Light Source ͑ALS͒ at Lawrence Berkeley National Laboratory. Measurement pressure was kept Ͻ3ϫ10−11 Torr, and data were recorded by Scienta R4000 analyzers at 15 K sample temperature. The total convolved energy and angle resolu- tions were 16 meV and 0.2° ͓i.e., Ͻ0.007 ͑Å−1͒ or Ͻ0.012͑1Å−1͒ for photoelectrons generated by 48 eV pho- tons͔, at which energy the cross-section for both surface state and bulk bands is strong. In Fig. 2 we show complimentary ARPES and SdH data on samples from the same batch with carrier density deter- ϫ 19 −3 mined by the Hall effect of ne =2.3 10 cm . The SdH FIG. 2. ͑Color online͒͑a͒ ARPES band dispersion on samples of reveals an anisotropic pocket of frequency 155 T, corre- Bi Se with carrier density 2.3ϫ1019 cm−3 ͑batch S4͒. Green lines sponding to a Fermi energy of around 155 meV above the 2 3 mark the Fermi level, the bottom of the conduction band, E−EF bottom of the conduction band ͑the band structure is not =−150 meV and the Dirac point, E−EF =−355 meV. ͑b͒ Due to parabolic at this filling and so we assume similar band- the quantization of the energy spectrum into Landau levels, oscilla- 12 structure parameters as Köhler characterizing similar car- tions appear in the magnetoresistance known as SdH oscillations. ͒ rier density samples of Bi2Se3 .
Details
-
File Typepdf
-
Upload Time-
-
Content LanguagesEnglish
-
Upload UserAnonymous/Not logged-in
-
File Pages5 Page
-
File Size-