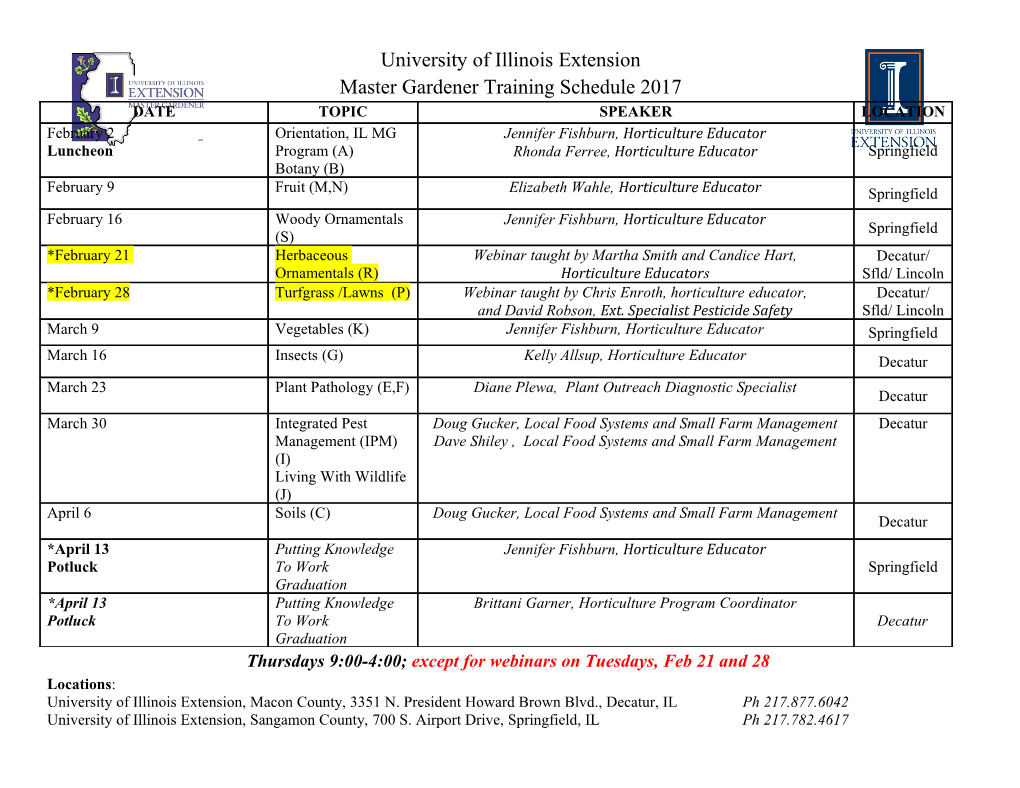
DNA Replication and Repair – Sep. 26-28, 2007 Dr. Jim Borowiec (MSB383A) (E-mail: [email protected]) I. DNA Replication A. Requirement for high-fidelity DNA replication B. Enzymology and mechanism of DNA synthesis 1. General description of replication process 2. Properties of DNA polymerases 3. Therapeutic nucleotides 4. Okazaki fragment synthesis Priming and processing 5. Other replication factors Sliding clamps, DNA helicases, etc. C. Initiation of DNA replication D. Telomeres II. DNA Repair A. Types of DNA damage Spontaneous and environmental B. Base excision repair DNA glycosylases, AP endonuclease C. Nucleotide excision repair E. coli UvrABC endonuclease, helicase II (uvrD) Human xeroderma pigmentosum (XP) complementation groups D. Mismatch repair E. coli mutS, mutH, mutL and determination of incorrect strand human hMSH2, hMLH1 and hereditary nonpolyposis colon cancer E. Defects in cell cycle checkpoints Ataxia Telangiestasia p53 mutation 1 Overview of DNA replication Telomere Centromere Telomere DNA chromosome Origin of DNA replication Specialized elements termed 'origins of DNA replication' occur many times on a chromosome Initiation of DNA replication from origin of replication generates structures termed 'DNA replication bubbles'. (One of many replication bubbles is shown) direction of fork movement Each replication bubble Nascent contains two 'DNA (daughter) strands replication forks' moving in opposite directions. (An enlarged view of one fork Parental strands is shown here) DNA replication complex (bound to fork) Action of DNA replication complex (acting on fork) + duplicates both parental strands 2 Continual Priming of Okazaki Fragments 3' 5' LEADING STRAND Nascent leading DNA 5' RNA primer 3' 3' Nascent lagging DNA FORK MOVEMENT 3' LAGGING STRAND 5' Priming New priming event 5' 3' Extension 5' Primer extension 3' Processing 5' Removal of RNA primer 3' and ligation Replication employs two different mechanisms to replicate the leading and lagging strands. DNA synthesis on the leading strand occurs in the same direction as fork movement, is continuous and generally highly processive. Lagging strand DNA synthesis occurs in the direction opposite to fork movement, is discontinuous and uses DNA polymerases with moderate processivity. 3 The DNA polymerase enzyme. The structure of various DNA polymerases have been solved by X-ray crystallography. These structures indicate that the ‘typical’ DNA polymerase resembles a human right hand, in which the thumb, palm, and fingers clasp the DNA. In this way, the DNA is properly positioned for association with the polymerase active site and catalytic efficiency is promoted. P incoming P 3' deoxyribonucleoside OH triphosphate 5' 3' P primer 5’ strand P P P OH template strand P P P P P P 3' 5' P P 2 Pi 5'’ 3' P P P P OH 5'-to-3' direction of chain growth P P P P P P 3' 5' Nascent strand synthesis by DNA polymerases occurs only in the 5’ to 3’ direction. Nucleotide incorporation utilizes two high-energy phosphate bonds, driving the reaction in the forward direction. 4 H H NORMAL C:G base pair N H N N H O O N N N H N H N BASE Cytosine O N (favored) (amino form) N H N H ABNORMAL C*:A base pair H BASE* N H (rare) H N H H N N N N N H O N N H O N Cytosine* N H (imino form) ↑ Anomalous base pairing can occur that involves rare tautomeric forms of the bases. In the example shown, when cytosine is in the rare imino form (C*), it can form a base pair with the common amino form of adenine (A). The rare form of adenine (A*) can also pair with the common form of cytosine (C). G*:T and G:T* base pairs can also form. Defective base insertion can be corrected either by DNA polymerase proofreading or mismatch repair. An important process required for high fidelity DNA replication is proofreading. DNA molecules with a mismatched nucleotide at the 3′ end of the primer strand (i.e., because of DNA polymerase misincorporation) are poor templates for continued DNA synthesis and usually cause stalling of the DNA polymerase. A 3′-to-5′ proofreading exonuclease associated with the polymerase enzyme removes unpaired nucleotides from the 3′ end of the nascent strand. The DNA polymerase can therefore act as a self-correcting enzyme to remove its own polymerization mistakes. 5 The activities of key replication enzymes are conserved in bacteria (E. coli) and eukaryotes (human). For example, the overall structure of the E. coli β protein and the human PCNA are similar, even though the gene sequences are divergent. 6 Polymerase name Function DNA polymerase α Primer function in DNA (pol α) replication Base excision repair pol β Mitochondrial DNA pol γ replication and repair DNA replication; nucleotide pol δ and base excision repair DNA replication; nucleotide pol ε and base excision repair Translesion synthesis? pol ζ Accurate translesion pol η synthesis DNA repair of crosslinks? pol θ Somatic hypermutation pol ι Error-prone repair pol κ Meiosis-associated DNA pol λ repair? Somatic hypermutation? pol µ Human DNA-directed DNA polymerases. Human cells, similar to prokaryotic and other eukaryotic cells, contain multiple DNA polymerases. The activities of these DNA polymerases differ to a certain extent because of the specific roles they play in DNA replication, repair, and recombination. The most important human DNA polymerases for general DNA replication are DNA polymerase α (which has an associated DNA primase activity) and DNA polymerase δ. DNA polymerase δ is the major DNA polymerase required for processive leading strand synthesis, but also functions to complete Okazaki strand synthesis on the lagging strand template. DNA polymerases α to ε have been known for a number of years and are fairly well characterized. Most of the other DNA polymerases have been identified only very recently, and the characterization of their functional role is ongoing. Note that the list is not comprehensive – additional DNA polymerases have been identified which are not included in this list. 7 Therapeutic nucleoside analogs 1. Anticancer O Cytosine H O-CH Converted to the nucleoside triphosphate. Inhibits 2 elongation of DNA synthesis by competing with dCTP H OH for DNA polymerase. Can act as a chain terminator - H H selectively kills growing cells. Antileukemic. OH H cytosine arabinoside (araC) 2. Antiviral A. Anti-HIV Converted to the nucleoside triphosphate. Selectively inhibits HIV reverse transcriptase activity (an essential O Thymine H O-CH HIV DNA polymerase that synthesizes the HIV DNA 2 strands from the RNA template). Acts as a chain H H terminator. H H N 3 H 3'-deoxy-3'-azidothymidine (AZT) O Inosine H O-CH2 Inosine is a precursor to purines guanine and adenine. H H Converted to the nucleoside triphosphate. Selectively H H inhibits HIV reverse transcriptase activity. Acts as a chain terminator. H H dideoxyinosine (ddI) B. Other Anti-Viral Guanine Potent inhibitor of herpes simplex viruses (HSV). H O-CH O Converted to the triphosphate. Inhibits activity of HSV 2 DNA polymerase while having lesser effect on cellular DNA polymerases. Acts as a chain terminator. H H H H Acyclovir 8 Okazaki fragment (OF) synthesis in human cells Norma lly occurs on lagging strand Two DNA polymeras es are used RNA primer synthesis by DNA primase (assoc. with DNA pol ") 5' end of previous OF Step 1. Priming DNA polym erases can not start nascent strand synthesis de novo. Need RNA priming by DNA primase 3' 5' assoc iated with DNA pol ". Primer FORK MO VEMENT LAG GING STRAND is ~10 nt in length. TEMP LATE iDNA synthesis DNA pol " by pol " Step 2. Synthesis of initiator DNA (iDNA). DNA pol " extends the RNA 3' 5' primer. The iDNA is ~35 nt in length, and acts as a DNA primer. DNA pol ! Synthesis of body of OF Nick in DNA (contains 3'-O H) by pol ! Step 3. Extension of iDNA. DNA pol ! (which has no priming activity) extends the iDNA. 3' 5' Extension continues ~200 nt to 5' end of previous OF. RNase H + nucl ease Step 4. Removal of RNA prim er. The RNA primer is removed by RNase H (which cleaves the RNA 3' 5' portion of a DNA-RNA hy brid). Most of the iDNA is also removed by a nuclease. Occurs while next OF is being syn thesized. DNA ligase Step 5. Ligation. In the final reaction, the OFs 3' 5' are joined 9 A Human B E. coli RNA previous Okazaki RNA previous Okazaki RPA primer fragment SSB primer fragment 1 3' 1 3' 3' 5' Lagging strand template 3' 5' Lagging strand template pol α/primase primase Priming and DNA 5' 5' RNA primer synthesis by 2 3' synthesis by 2 3' 3' 5' 3' 5' primase pol α/primase PCNA/pol δ pol III holoenzyme 5' 3' Recognition by PCNA 5' 3' Recognition by pol III 3 3' 5' and DNA pol 3 δ 3' 5' holoenzyme PCNA/pol δ 4 5' 3' 4 5' 3' Elongation 3' 5' Elongation 3' 5' RNase H RNase H 5 5' 3' Removal of RNA primer 5 5' 3' Removal of RNA primer 3' 5' by RNase H/nuclease 3' 5' by RNase H/pol I 6 5' 3' 6 5' 3' 3' 5' Gap-filling 3' 5' Gap-filling DNA ligase DNA ligase 7 5' 3' Joining of Okazaki 7 5' 3' Joining of Okazaki 3' 5' fragments 3' 5' fragments Eukaryotic and prokaryotic replication utilize a generally similar mechanism of lagging strand synthesis. In the case of the eukaryotic replication, the DNA polymerase alpha/DNA primase (pol α/primase) first makes a short RNA/DNA hybrid, which DNA polymerase delta (pol δ) then extends. Processing enzymes including RNase H and DNA ligase join the Okazaki fragments.
Details
-
File Typepdf
-
Upload Time-
-
Content LanguagesEnglish
-
Upload UserAnonymous/Not logged-in
-
File Pages21 Page
-
File Size-