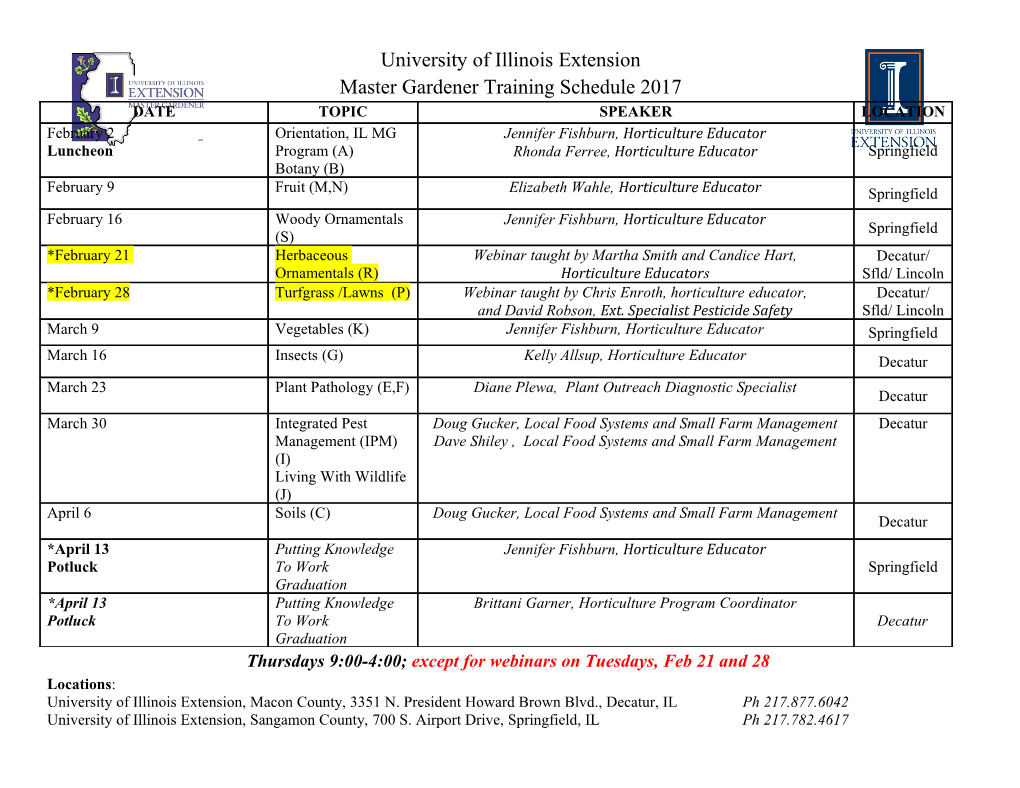
Journal of Experimental Botany, Vol. 65, No. 11, pp. 2835–2845, 2014 doi:10.1093/jxb/ert379 Advance Access publication 12 November, 2013 REVIEW PAPER Light perception and signalling by phytochrome A J. J. Casal1,2,*, A. N. Candia1 and R. Sellaro1 1 IFEVA, Facultad de Agronomía, Universidad de Buenos Aires and CONICET, 1417 Buenos Aires, Argentina 2 Fundación Instituto Leloir, Instituto de Investigaciones Bioquímicas de Buenos Aires–CONICET, C1405BWE Buenos Aires, Downloaded from https://academic.oup.com/jxb/article-abstract/65/11/2835/2877369 by guest on 27 February 2019 Argentina * To whom correspondence should be addressed. E-mail: [email protected] Received 30 August 2013; Revised 11 October 2013; Accepted 14 October 2013 Abstract In etiolated seedlings, phytochrome A (phyA) mediates very-low-fluence responses (VLFRs), which initiate de-eti- olation at the interphase between the soil and above-ground environments, and high-irradiance responses (HIR), which complete de-etiolation under dense canopies and require more sustained activation with far-red light. Light- activated phyA is transported to the nucleus by FAR-RED ELONGATED HYPOCOTYL1 (FHY1). The nuclear pool of active phyA increases under prolonged far-red light of relatively high fluence rates. This condition maximizes the rate of FHY1–phyA complex assembly and disassembly, allowing FHY1 to return to the cytoplasm to translocate further phyA to the nucleus, to replace phyA degraded in the proteasome. The core signalling pathways down- stream of nuclear phyA involve the negative regulation of CONSTITUTIVE PHOTOMORPHOGENIC 1, which tar- gets for degradation transcription factors required for photomorphogenesis, and PHYTOCHROME-INTERACTING FACTORs, which are transcription factors that repress photomorphogenesis. Under sustained far-red light activa- tion, released FHY1 can also be recruited with active phyA to target gene promoters as a transcriptional activa- tor, and nuclear phyA signalling activates a positive regulatory loop involving BELL-LIKE HOMEODOMAIN 1 that reinforces the HIR. Key words: CONSTITUTIVE PHOTOMORPHOGENIC 1 (COP1), high-irradiance response (HIR), nuclear translocation, phytochrome, PHYTOCHROME INTERACTING FACTOR (PIF), very-low-fluence response (VLFR). Introduction Plant phytochromes are a family of red/far-red light photore- phyA–phyA homodimers but no heterodimers with other ceptors that bear a linear tetrapyrrole chromophore attached family members (Sharrock and Clack, 2004). through a cysteine residue to their N-terminal domain (Vierstra and Zhang, 2011). This review is focused on phy- Subcellular localization of phyA tochrome A (phyA), a key member of the family with spe- cific and shared functions. Phytochromes are synthesized in In darkness, phyA is dispersed in the cytoplasm (Kircher et al., the inactive Pr form. Pr absorbs maximally in red light and, 2002; Toledo-Ortiz et al., 2010). There is nuclear phyB but no after excitation, relaxes into the active, Pfr form. In turn, Pfr detectable nuclear phyA in dark-grown seedlings, and in chi- has its maximum absorbance in far-red, which back-converts meric phyA–phyB phytochromes this differential pattern is the molecule into Pr. Due to the partial overlap between Pr defined by the C-terminal domain (Oka et al., 2012). Nuclear and Pfr absorption spectra (Fig. 1, inset), far-red light is able localization of phyA can be detected after 5 min of irradia- to transform a small proportion of the Pr molecules into tion with red or far-red light that transform part of the Pr Pfr. phyA monomers have a mol. wt of ~120 kDa and form pool into Pfr (Toledo-Ortiz et al., 2010). phyA lacks a known © The Author 2013. Published by Oxford University Press on behalf of the Society for Experimental Biology. All rights reserved. For permissions, please email: [email protected] 2836 | Casal et al. Downloaded from https://academic.oup.com/jxb/article-abstract/65/11/2835/2877369 by guest on 27 February 2019 Fig. 1. Signalling by phyA under VLFR and HIR conditions. Three major modules are represented: the phyA perception module (Rausenberger et al., 2011), the COP1 signalling pathway, and the PIF signalling module. Note that the HIR and VLFR differ in the perception module (Rausenberger et al., 2011) and in the regulatory loops connected to the downstream signalling modules (Shen et al., 2009; Staneloni et al., 2009; Yang et al., 2009; Chen et al., 2012). nuclear localization signal, and its nuclear presence depends ubiquitin E3 ligases (Quint et al., 2005; Debrieux et al., primarily on FAR-RED ELONGATED HYPOCOTYL1 2013) and, under certain conditions, on CONSTITUTIVE (FHY1) and secondarily on its homologue FHY1-LIKE PHOTOMORPHOGENIC1 (COP1) ubiquitin E3 ligase (Seo (FHL) (Hiltbrunner et al., 2005, 2006). The nuclear localiza- et al., 2004; Debrieux et al., 2013). Under light/dark cycles, tion signal and the phyA-interaction domain of FHY1 (pre- phyA accumulates during the night and becomes rapidly sent in the N- and the C-terminus, respectively) are conserved degraded during the day (Sharrock and Clack, 2002), despite in different species and are sufficient for FHY1 to transport the fact that PHYA promoter activity is maximal during the phyA to the nucleus (Genoud et al., 2008). Under daily pho- light phase due to the control by the circadian clock (Tóth toperiods of far-red light, the number of nuclei with speckles et al., 2001). phyA purified from dark-grown oat seedlings is containing phyA is higher during daytime than during the phosphorylated (Lapko et al., 1999), and purified recombi- night, but the levels increase before the beginning of the day, nant oat phyA autophosphorylates (Han et al., 2010). When arguing in favour of a circadian control of phyA localization expressed in Arabidopsis, mutations or deletions involving (Kircher et al., 2002). phosphorylation sites present at the N-terminal extension of phyA enhance the stability of oat phyA (Han et al., 2010) phyA abundance but reduce the stability of Arabidopsis phyA (Trupkin et al., 2007). These discrepancies might reflect the use of oat versus In dark-grown seedlings, phyA is the most abundant member Arabidopsis phyA in the Arabidopsis background. of the phytochrome family (Sharrock and Clack, 2002). Light down-regulates the abundance of phyA at transcriptional and Pfr to Pr thermal reversion post-transcriptional levels. Compared with full darkness, light perceived by phyA or Dark reversion is the light-independent conversion of Pfr phyB represses the expression of the PHYA gene (Quail, into Pr and reveals the instability of Pfr likely when it is 1994; Cantón and Quail, 1999). This repression is accompa- forming a heterodimer with Pr. This process affects the abun- nied by a rapid decrease in H3K4me3 and H3K9/14ac activat- dance of Pfr but not that of total phyA. In Arabidopsis only ing chromatin marks and a rapid increase in the H3K27me3 some accessions show thermal reversion of phyA Pfr, and repressive mark at the PHYA promoter (Jang et al., 2011). the source of this natural variation is extragenic to PHYA, These chromatin modifications are mediated in part by phyB indicating that the cellular environment strongly affects Pfr (Jang et al., 2011). stability (Hennig et al., 1999; Eichhenberg et al., 2000). While phyA is stable in the Pr form, the half-life of phyA Pfr is 0.5–2 h due to phyA ubiquitination and 26S protea- some degradation (Clough and Vierstra, 1997; Hennig Molecular and cellular dynamics of phyA et al., 1999). Both, nuclear and cytoplasmic pools are and the perception of light signals degraded in the proteasome, but nuclear degradation is faster (Debrieux and Fankhauser, 2010; Toledo-Ortiz et al., 2010). The analysis of the relationship between light input and Degradation of phyA depends primarily on CULLIN1-based physiological outputs under controlled conditions has Signalling by phyA | 2837 traditionally defined three modes of photobiological action be interpreted as a repeated LFR of phyB (Mazzella et al., of phytochromes: the very-low-fluence responses (VLFRs), 1997). Only a very small proportion of the effect of con- the low-fluence responses (LFRs), and the high-irradiance tinuous far-red can be replaced by hourly pulses of far-red responses (HIRs) (Casal et al., 1998). These photobiologi- (Mancinelli, 1994). When dark-grown seedlings are exposed cal modes of action partially reflect the contribution of dif- to pulses of far-red light of different frequencies, two phases ferent members of the phytochrome family to physiological of response can be distinguished (Casal et al., 2000; Zhou responses. et al., 2002). The first phase saturates with a frequency of one pulse every 120 min and corresponds to the contribution The VLFR mediated by phyA of the VLFR. The second phase is observed at frequencies above one pulse every 30 min, and that represents the specific When imbibed seeds are exposed to a pulse of red light, the contribution of the HIR. induction of germination above that of dark controls often VLFRs and HIRs can be genetically dissected at the level shows a biphasic response to the fluence of the light pulse. of the phyA molecule itself. The phyA-302 allele, in which Downloaded from https://academic.oup.com/jxb/article-abstract/65/11/2835/2877369 by guest on 27 February 2019 The first phase, which typically saturates at 1 μmol. m–2 is Glu777 (a residue conserved in angiosperm phytochromes) the VLFR, and the second phase, observed at higher fluen- changed to lysine in the PAS2 motif of the C-terminal cies is the LFR (Cone et al., 1985; Casal et al., 1998). The domain, retains VLFRs but lacks HIRs (Yanovsky et al., VLFR is mediated by phyA and is therefore absent in the 2002). This mutant fails to form phyA nuclear speckles, and phyA mutant (Botto et al., 1996; Shinomura et al., 1996). The has slightly reduced phyA levels in darkness, compensated by VLFR is triggered by the transformation of a small propor- enhanced phyA stability under far-red light.
Details
-
File Typepdf
-
Upload Time-
-
Content LanguagesEnglish
-
Upload UserAnonymous/Not logged-in
-
File Pages11 Page
-
File Size-