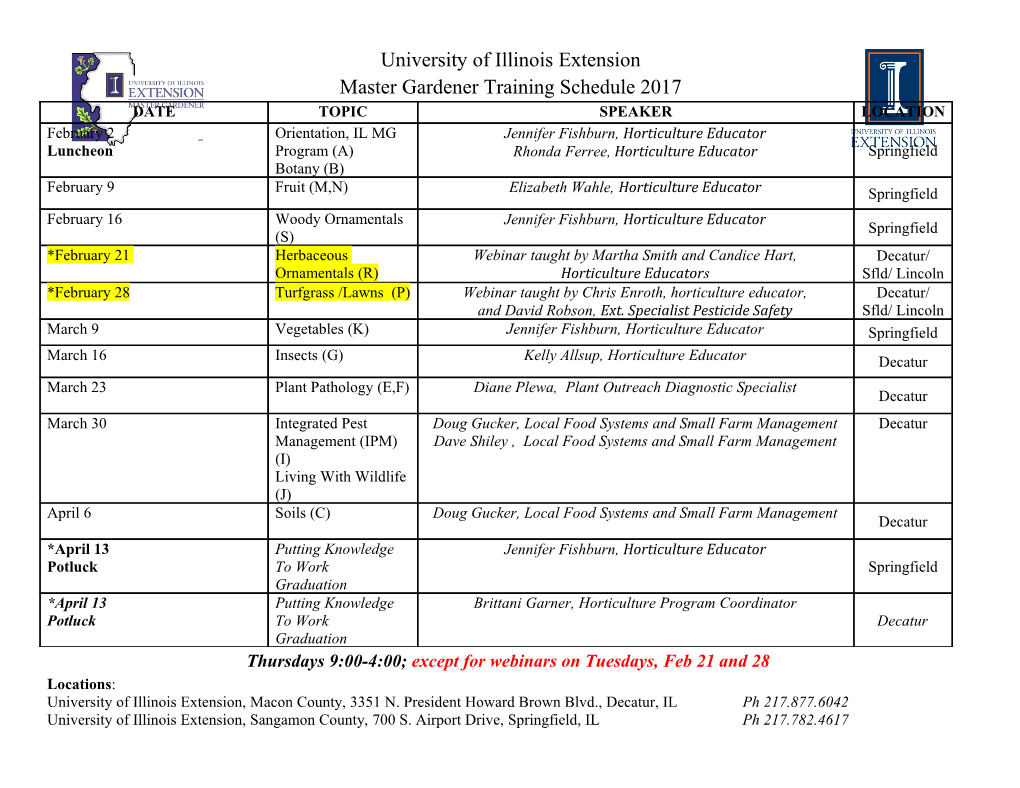
HIGH-PRESSURE MOSSBAUER RESONANCE STUDIES OF THE CONVERSION OF Fe(III) TO Fe(II) IN FERRIC HALIDES* BY G. K. LEWIS, JR., AND H. G. DRICKAMER DEPARTMENT OF CHEMISTRY AND CHEMICAL ENGINEERING AND MATERIALS RESEARCH LABORATORY, UNIVERSITY OF ILLINOIS, URBANA Communicated July 24, 1968 In several recent papers1-3 it has been shown by high-pressure Mbssbauer studies that ferric ion reduces to ferrous ion at high pressure, and that this is an essentially reversible process. In this paper we present data on FeCl3, FeBr3, and KFeCl4 to 175 kilobars (kb). FeCl3 and FeBr3 each have an octahedral arrangement of the Cl- ions around the iron, while the FeCl4- ion exhibits tetrahedral symmetry. The compounds were synthesized from iron enriched to 77 per cent in Fe57 by using methods available in the literature.4-8 Since FeCl3 and FeBr3 are extremely hydroscopic and KFeCl4 is moderately so, all handling was done in a dry box in a dry argon atmosphere. Each material gave the appropriate X-ray spectrum upon analysis. The high-pressure M6ssbauer techniques have been previously described.9 At each pressure the spectrum was run until at least 200,000 counts had accumulated in each channel. (Where the effect was over 20 per cent only 150,000 counts per channel were obtained.) The spectra were computer-fit with pairs of Lorentzian peaks. A minimum of five loads was run on each substance at 2940K. In addition, at several pressures isobars were run to 4180K, and several high-temperature isotherms were obtained. As discussed in detail in reference 1, it is easy to identify high-spin ferrous and ferric states from M6ssbauer spectra. The ferrous state exhibits a rela- tively low electron density with isomer shifts in the range 1.2-1.4 mm/sec below bec iron and high quadrupole splitting (2.0-3.0 mm/sec). The ferric state ex- hibits isomer shifts in the range 0.3-0.5 mm/sec below iron and small quadrupole splitting (0-0.7 mm/see) caused entirely by a lack of cubic symmetry at the Fe(III) center. It is difficult to determine highly precise values for the change of isomer shift and quadrupole splitting with pressure on systems which exhibit a large change in the ferrous-ferric ratio, as these do (see below). However, Table 1 lists the average values for these systems. Both ferric and ferrous ions show an increase in electron density at the nucleus with increasing pressure, as do most nonreacting systems.1 The major cause is probably changes in the 3d-3s shielding as discussed in reference 1. There is a relatively large increase in quadrupole splitting for the ferric systems, whereas the ferrous quadrupole splitting is relatively independent of pressure. This is not unreasonable in first order as the splitting for Fe(III) results directly from lattice distortions (qiat), while the Fe(II) splitting is due to asymmetric distribution of the 3d electron (qvai). The most striking feature of the spectra is the large conversion of the ferric ion to the ferrous state even at pressures as low as 10 kb. Figure la-d shows typical spectra. Figure 2 exhibits a plot of ln K versus ln P, where K = CII/CIII is 414 Downloaded by guest on September 30, 2021 VOL. 61, 1968 CHEMISTRY: LEWIS AND DRICKAMER 415 z o .000 a- cr .030 0 aI .060 (a) 3-5 kbar < .090 z 0 p .120 c: .150 LL FIG. la, b.-Typical spectra, FeC1h. (a) 3-5 kb; (b) 11 kb. .000 z °.050 0- ° .100 (a Ji .150 z °.200 ( .250 -2.0 -1.0 0 1.0 2.0 3.0 4.0 DOPPLER VELOCITY IN MM/SEC FIG. ic, d.-Typical spectra, FeCh3. (c) 127 kb; (d) after release of pressure. Downloaded by guest on September 30, 2021 416 CHEMISTRY: LEWIS AND DRICKAMER PROC. N. A. S. TABLE 1. Isomer shifts* and quadrupole splittingt of the ferric halides. Pressure (kb) FeCl3 FeBr3 KFeC14 Fe(III) isomer shift 4 0.35 0.35 0.32 40 0.32 - - 100 0.27 0.32 0.31 125 0.26 - - Fe(II) isomer shift 10 1.30 1.30 1.28 40 1.27 - 100 1.24 1.22 1.26 125 1.22 - - Fe(III) quadrupole splitting 4 0.88 0.80 0.50 40 1.20 0.95 0.80 100 1.40 1.05 1.10 125 1.49 1.20 Fe(II) quadrupole splitting 10 2.15 2.30 1.95 100 2.10 2.17 1.95 * Mm/sec relative to bcc iron metal. t Mm/sec. an equilibrium constant. It is seen that these data follow quite accurately the form: K = APB, (1) where A and B are constants. An analysis of the data of Champion'0 and of Vaughan" shows equally good agreement with this form of equation. The constants are included in Table 2. It may be mentioned parenthetically that the data for a variety of other ferric systems now under study in this laboratory appear to follow this same relation. From Table 2 it may be seen that B - 0.5 for more ionic compounds and is measurably larger for more covalent materials. It was difficult to obtain good high-temperature data for the halide systems because of the high conversion, but, within our accuracy, B appears to be independent of temperature for these materials. This is not universally true. Figure 3 shows the results of isobars obtained at 11 kb. The heats of reaction are listed in Table 3. They increase measurably with increasing temperature for FeCl3 and FeBr3. Nor- mally samples were diluted with boron, but no significant difference in behavior was observed for samples diluted with aluminum oxide or with boron carbide. It should be pointed out that what is observed is an equilibrium phenomenon, not the result of slow kinetics. Typical runs at a single pressure took 8-48 hours, and consecutive runs at the same pressure showed no change in con- version. However, when the pressure was increased, a definite increase in con- version was noted in the spectrum displayed on the oscilloscope as soon as enough counts were available to define a spectrum-usually within five minutes or so. From a molecular standpoint, there are two questions to be answered: (1) How are the electronic levels of Fe(III) lowered with pressure vis-A-vis the ligand levels to give thermal electron transfer? Downloaded by guest on September 30, 2021 VOL. 61, 1968 CHEMISTRY: LEWIS AND DRICKAMER 417 6.0 4.0 - FeC0 3 2.0- .0 0.6- 0.2 0.1. 1 5 10 20 30 60 100 200 P(kbor) FIG. 2.-Ln equilibrium constant versus In pres- sure, FeC13, FeBr3, and KFeC14. (2) Once this lowering has taken place, why do we not observe complete re- duction of the ferric ion within a short pressure range? It is straightforward to identify the most probable energy levels involved. For an octahedral complex (see Ballhausen and Gray,'2 p. 103) the transfer must be from the ligand nonbonding t2. level to the predominantly metal anti- bonding t2,. For the tetrahedral case, the corresponding levels are the tj(7r) and e(7r). Broad charge transfer peaks are observed in most such complexes at 3-6 ev, with tails extending sometimes through the visible and even into the infra- red region of the spectrum.'3 One can imagine at least two mechanisms which could contribute to a red shift of these peaks (i.e., a lowering of the t2. t20 energy difference). The bond- ing for ionic complexes is largely a bonding, but it is possible for the t2, orbital to bond with the lr* orbitals of the ligand.'4 For these complexes one expects a markedly smaller r-ir overlap than u-a- overlap at 1 atmosphere, and with increasing pressure one would anticipate a larger increase in the former than in the latter. This overlap should tend to stabilize the t2g orbitals as they are bonding with respect to the ligand -r* orbitals. The reduction of Fe(III) ion takes place with a wide variety of ligands in various symmetries, so the possible causes, including ir bonding, must be independent of the details of structure. As a prototype, the Fe-Cl system was analyzed in both octahedral and tetra- hedral symmetry. Group overlap calculations were made as a function of interatomic distance in accordance with the procedures outlined in reference 12 and in references contained therein. Clementi's1' wave functions were used. Figure 4 shows the change in 7r and a- overlap with Fe-Cl distance for FeI+-Cl- in octahedral symmetry. Calculations for tetrahedral symmetry and for Fe'+- Cl- did not differ qualitatively. Indeed, the wr overlap increases faster than the a, which should be a stabilizing factor. However, the difference is not great Downloaded by guest on September 30, 2021 418 CHEMISTRY: LEWIS AND DRICKAMER PROC. N. A. S. TABLE 2. Parameters A and B for K = AP*. Compound A B FeCli 0.265 0.564 FeBr3 0.076 0.426 KFeCh4 0.091 0.497 Ferric phosphate1 0.787 0.457 Ferric citrate'0 0.112 0.350 Phosphate glass* 0.486 0.312 KFe(CN)6'0 0.109 2.06 Ferric acetate (3820K)* 0.22 X 10-6 3.05 Ferric acetate (4180K)* 0.022 0.986 * These data will be discussed elsewhere. enough to be a major factor in any large red shift of the charge transfer peak. The-second factor that would tend to decrease the tku -a tag energy difference is the radial spreading of the 3d orbitals with pressure.
Details
-
File Typepdf
-
Upload Time-
-
Content LanguagesEnglish
-
Upload UserAnonymous/Not logged-in
-
File Pages8 Page
-
File Size-