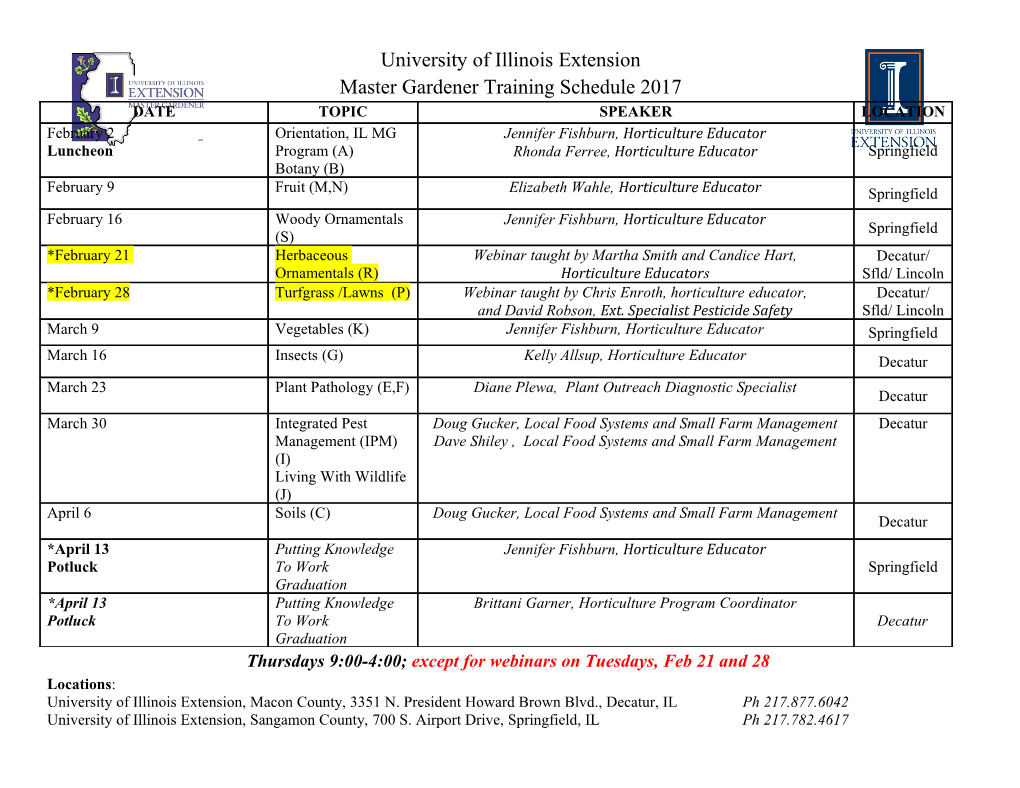
Medical Aspects of Harsh Environments, Volume 2 Chapter 32 PRESSURE CHANGES AND HYPOXIA IN AVIATION R. M. HARDING, BSC, MB BS, PHD* INTRODUCTION THE ATMOSPHERE Structure Composition THE PHYSIOLOGICAL CONSEQUENCES OF RAPID ASCENT TO ALTITUDE Hypoxia Hyperventilation Barotrauma: The Direct Effects of Pressure Change Altitude Decompression Sickness LIFE-SUPPORT SYSTEMS FOR FLIGHT AT HIGH ALTITUDE Cabin Pressurization Systems Loss of Cabin Pressurization Personal Oxygen Equipment SUMMARY *Principal Consultant, Biodynamic Research Corporation, 9901 IH-10 West, Suite 1000, San Antonio, Texas 78230; formerly, Royal Air Force Consultant in Aviation Medicine and Head of Aircrew Systems Division, Department of Aeromedicine and Neuroscience of the UK Centre for Human Science, Farnborough, Hampshire, United Kingdom 984 Pressure Changes and Hypoxia in Aviation INTRODUCTION The physiological consequences of rapid ascent and life-support engineers has established reliable to high altitude are a core problem in the field of techniques for safe flight at high altitudes, as demon- aerospace medicine. Those who live and work in strated by current atmospheric flight in all its forms, mountain terrain experience a limited range of al- military and civilian, from balloon flights to sail planes titudes and have time to adapt to the hypoxia ex- to supersonic aircraft and spacecraft. Although reli- perienced at high terrestrial elevations. In contrast, able cabin pressurization and oxygen delivery systems flyers may be exposed to abrupt changes in baro- have greatly reduced incidents and accidents due to metric pressure and to acute, life-threatening hy- hypoxia in flight, constant vigilance is required for poxia (see also Chapter 28, Introduction to Special their prevention. Environments). The aims of this chapter are, therefore, to present a In 1875, a landmark balloon flight to 28,820 ft ended distillation of our current comprehension of the phys- in tragedy when the three young French aeronauts ics and physiology of rapid ascent to high altitude and on board failed to use their supplemental oxygen ef- to describe the technology required to support human fectively; Tissandier survived, but his colleagues, Sivel existence in that most hostile of environments. To this and Crocé-Spineli, became the first known fatalities end, the chapter briefly characterizes the atmosphere due to in-flight hypoxia. In early aviation, too, the lack within which flight takes place, then describes nor- of oxygen took a regular toll of both lives and aircraft; mal physiological responses to acute hypobaria and many military crewmembers were killed by hypoxia, hypoxia, and finally describes the life-support systems and the performance of many more was significantly that enable flyers to operate safely at high altitudes. impaired in flight. Historic ascents over many years Military requirements drove much of the early re- demonstrated that even with inhalation of 100% oxy- search in aviation physiology as well as the develop- gen, unpressurized flight above 42,000 ft was imprac- ment of robust life-support systems. Prominent cen- tical because of the effects of hypoxia and extreme ters for aviation altitude research included the US Air cold. It was found that humans cannot adapt to hy- Force School of Aerospace Medicine, San Antonio, poxia in flight but must instead be provided with life- Texas; the now-defunct Royal Air Force Institute of support systems that (a) maintain physiological Aviation Medicine, Farnborough, Hampshire, En- normoxia under routine operating conditions and (b) gland; and various universities whose hypobaric protect from significant hypoxia in emergencies. chambers were supported with military research Pioneering research by physicians, physiologists, funds. THE ATMOSPHERE Earth’s atmosphere is vital to our existence: it pro- life at the surface, but high-altitude flight can produce vides a moderate temperature environment at the significant cumulative radiation exposure for flyers. surface, a protective barrier against the effects of ra- diation, and the oxygen needed for the release of bio- Structure logical energy. Flyers depart from the safety of this surface cocoon at their peril. The outer limit of the atmosphere is determined The physical characteristics of the atmosphere are by two opposing factors: solar heating tends to ex- a complex product of solar heating, ionizing radia- pand gases from the outer atmosphere into the sur- tion, and ozone formation (Figure 32-1). The upper rounding vacuum of space, while gravity tends to pull atmosphere reflects some solar radiation and absorbs the gases toward Earth’s surface. The structure of the the rest, re-radiating infrared energy into the lower atmosphere is conventionally described in terms of atmosphere and thence to Earth’s surface. This “green- several concentric shells with differing thermal pro- house” effect ensures that Earth’s surface is warmer files and other characteristics: than it would be if it received only direct solar heating. High-energy particulate material (cosmic radiation) • The troposphere (0–40,000 ft) is characterized continuously bombards the atmosphere. This primary by a steady decrease in temperature with ionizing radiation collides with atoms in the upper altitude, the presence of varying amounts atmosphere at high velocity to create secondary ra- of water vapor, and the occurrence of large- diation. Fortunately, the atmosphere further reduces scale turbulence (weather). This is the realm the level of this radiation so that it has little effect on of most conventional aviation, including 985 Medical Aspects of Harsh Environments, Volume 2 Exo- 800 sphere 700 iles m 600 435 Thermo- sphere 500 400 300 300,000 ft 200 100 250,000 ft Barometric Pressure (mmHg) Meso- 0 sphere 0 10 20 30 40 50 60 70 80 90100 Altitude Altitude (ft x 1,000) 200,000 ft Stratopause Fig. 32-2. The exponential relation of barometric pres- sure to altitude. Note that at 18,000 ft (380 mm Hg), baro- 150,000 ft Strato- metric pressure is half that at sea level. sphere Ozono- Ozonosphere sphere 100,000 ft ture approaches absolute zero, extends be- Tropopause yond the atmosphere to the vacuum of space. 50,000 ft Tropo- Mt Everest sphere 0 ft The pressure exerted by the atmosphere, termed –60 –40 –20 0 20 0510 barometric pressure (PB), falls exponentially with al- Temperature ( C) Ozone (ppmv) ° titude (Figure 32-2), producing a proportional de- crease in the partial pressures of oxygen and other Fig. 32-1. The structure of the atmosphere and the rela- constituent gases. The International Civil Aviation tion of altitude to temperature and ozone concentration. Organization’s (ICAO’s) Standard Atmosphere is an Note that at the top of Mount Everest, the highest point agreed description of the relation among PB, tem- on Earth (29,028 ft), the temperature is about –40°C and perature, and altitude at a latitude of 45° north ozone concentration is about 1 ppmv. For the next 125,000 1 ft the temperature becomes more moderate while ozone (Table 32-1). This description forms the basis for becomes more, then again less, concentrated. The tem- calibration of pressure-measuring flight instru- perature at the top of Mount Everest, –40°C, is not ments and allows precise comparisons between the reached again until about 100,000 ft. Adapted with per- performances of different aircraft and aircraft sys- mission from Harding RM. The Earth’s atmosphere. In: tems. The measurement of physiological variables Ernsting J, King P. Aviation Medicine. 2nd ed. London, such as volume and mass of gas, gas flow, and meta- England: Butterworths; 1988: 5. bolic rate, as well as the related specifications for life- support systems, are profoundly affected by changes in body temperature, PB, and saturation of water va- balloon flights. por in the lungs. The conditions of measuring physi- • The stratosphere (40,000–160,000 ft) includes ological variables at altitude are summarized in Ex- a lower isothermal layer, above which tem- hibit 32-1. (Related information on the larger changes perature increases with altitude. Ozone is in PB associated with diving is found in Chapter 30, formed in the stratosphere, and flight in this Physics, Physiology, and Medicine of Diving.) region is generally limited to military air- craft. Composition • The mesosphere (160,000–260,000 ft) exhib- its a rapid decline in temperature with The atmosphere is made up of a remarkably con- altitude. stant mixture of nitrogen and oxygen, with traces of • The thermosphere, also called the ionosphere other gases (Table 32-2). In addition, the lower tropo- (260,000 ft to ~ 435 mi [700 km, the outer limit sphere may contain significant amounts of carbon of the atmosphere]), exhibits extreme tempera- dioxide and toxic gases, reflecting human activity and tures, which vary with solar activity. natural phenomena such as volcanic eruptions. It may • The exosphere (≥ 435 mi), where the tempera- also contain increased quantities of water vapor, de- 986 Pressure Changes and Hypoxia in Aviation TABLE 32–1 INTERNATIONAL CIVIL AVIATION ORGANIZATION STANDARD ATMOSPHERE Altitude Pressure Temperature Altitude Pressure Temperature (ft) (m) (mm Hg) (°C) (ft) (m) (mm Hg) (°C) 00 760 +15.0 25,000 7,620 282 –34.5 1,000 305 733 +13.0 30,000 9,144 226 –44.4 2,000 610 706 +11.0 35,000 10,668 179 –54.2 3,000 914 681 +9.1 40,000 12,192 141 –56.5 4,000 1,219 656 +7.1 45,000 13,716 111 –56.5 5,000 1,525 632 +5.1 50,000 15,240 87.3 –56.5 6,000 1,829 609 +3.1 55,000 16,764 68.8 –56.5 7,000 2,134 586 +1.1 60,000 18,288 54.1 –56.5 8,000 2,438 565 –0.9 65,000 19,812 42.3 –56.5 9,000 2,743 543 –2.8 70,000 21,336 33.3 –55.2 10,000 3,048 523 –4.8 80,000 24,384 20.7 –52.1 15,000 4,572 429 –14.7 90,000 27,432 13.0 –49.1 20,000 6,096 349 –24.6 100,000 30,480 8.2 –46.0 Adapted with permission from International Civil Aviation Organization.
Details
-
File Typepdf
-
Upload Time-
-
Content LanguagesEnglish
-
Upload UserAnonymous/Not logged-in
-
File Pages30 Page
-
File Size-