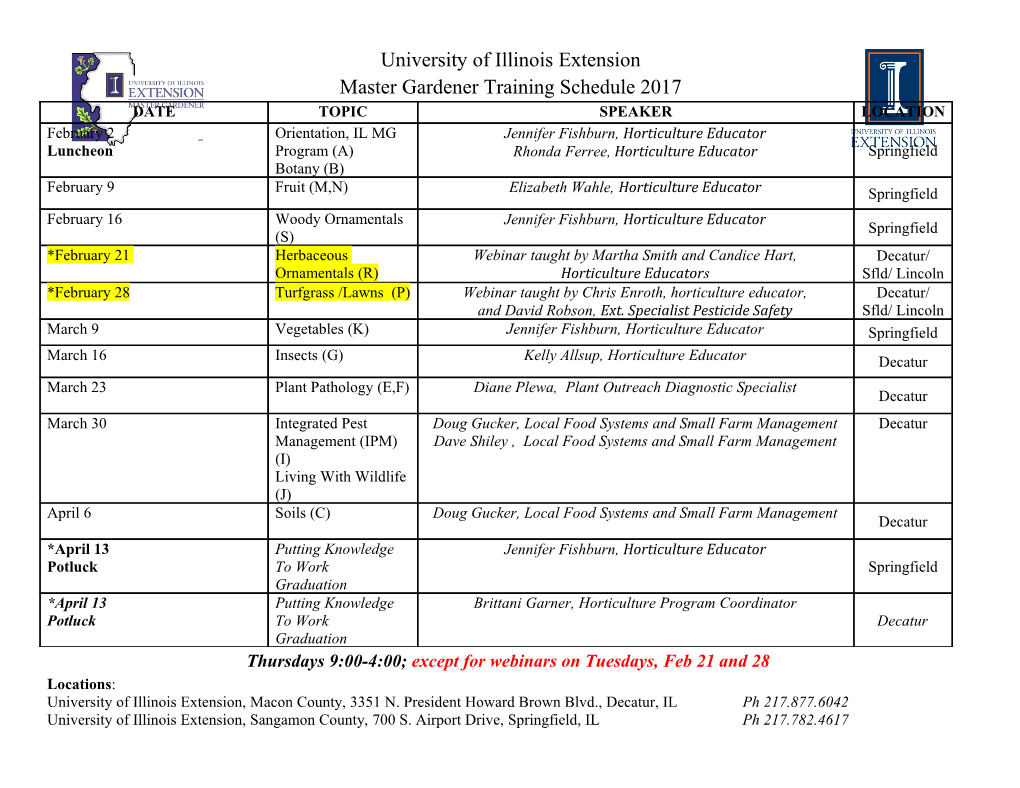
Resolution Roll Call: Optical Sectioning System Overviews Principles and Comparisons of Fluorescence-Based High-Resolution Imaging Modalities Matt Curtis 3D Imaging Specialist Jamie Scott Account Manager University of Illinois January 2019 Seite Carl Zeiss Microscopy 1/28/2019 1 Outline of Discussion High Resolution Optical Sectioning 1 Basic Aspects of Resolution in X, Y, and Z 2 Modalities for Enhancing Axial Resolution 3 Advanced Approaches for Exceeding the Resolution Limit 4 Summary of Techniques 5 Questions Carl Zeiss Microscopy 1/28/2019 2 Outline of Discussion High Resolution Optical Sectioning 1 Basic Aspects of Resolution in X, Y, and Z 2 Modalities for Enhancing Axial Resolution 3 Advanced Approaches for Exceeding the Resolution Limit 4 Summary of Techniques 5 Questions Carl Zeiss Microscopy 1/28/2019 3 Aspects of Resolution Defining the Limits • Ernst Abbe deduced the “resolution limit” of lenses at Carl Zeiss’ Jena workshop in 1873 - Expanded concepts of optical imaging using wave properties of light • Resolution defined as the minimum distance (d) necessary to distinguish two point-like objects in lateral space d Carl Zeiss Microscopy 1/28/2019 4 Aspects of Resolution Historical Context • Thomas A. Edison’s electric lamp (1880) Carl Zeiss Microscopy 1/28/2019 5 Aspects of Resolution Defining the Limits • Numerical aperture (NA) is the defining characteristic for resolution 퐍퐀 = 풏 퐬퐢퐧 훂 C-APOCHROMAT n = refractive index of immersion media (describes how light propagates relative to vacuum) 40x/1.2 W DIC α = half angle; acceptance cone of light • Resolution criteria is dependent on both wavelength and NA (not magnification) 훌 ퟎ. ퟓ 훌 퐝 = = 퐱퐲 ퟐ(풏 퐬퐢퐧 훂 ) 퐍퐀 Carl Zeiss Microscopy 1/28/2019 6 Aspects of Resolution Comparing Numerical Aperture Object Image • All other things constant, changes in NA = 1.4 α = 67° NA influence the 0.3 µm image resolution NA = 1.2 • In practical terms, this α = 52° has significance in all applications involving structural NA = 0.9 or molecular α = 36° localization • One object/puncta or NA = 0.5 actually several? α = 20° Carl Zeiss Microscopy 1/28/2019 7 Aspects of Resolution Comparison of Example Objectives Magnification NA Resolution (XY) 4x 0.10 2.75 μm 4x 0.20 1.375 μm 10x 0.25 1.10 μm 10x 0.45 0.61 μm 20x 0.40 0.69 μm 20x 0.75 0.37 μm 40x 0.65 0.42 μm 40x 1.40 0.20 μm 63x 0.75 0.37 μm 63x 1.30 0.25 μm 100x 1.25 0.22 μm 100x 1.40 0.20 μm Carl Zeiss Microscopy 1/28/2019 8 Aspects of Resolution Comparison of Example Objectives Plan-Apochromat 63x/1.4 Oil Alpha-Fluar 100x/1.45 Oil • Even small increases in NA can yield key improvements in imaging Carl Zeiss Microscopy 1/28/2019 9 Aspects of Resolution Limits in the Z Dimension Object Image • The same principles of ퟎ. ퟓ 훌 resolution extend into 퐝 = 퐱퐲 퐍퐀 the axial (Z) dimension - For widefield imaging lateral resolution system, minimum y (XY) limit ~ 200 nm x resolved distance in Z z is larger than in XY ퟐ훌 WHY WORSE IN Z? 퐝 = 퐙 퐍퐀ퟐ • Out-of-focus light interference in sample axial resolution • Non-symmetrical (Z) limit ~ 550 nm wavefront from lens along the optical axis Carl Zeiss Microscopy 1/28/2019 10 Aspects of Resolution Comparison of Example Objectives Magnification NA Resolution (XY) Resolution (Z) 4x 0.10 2.75 μm 110 μm 4x 0.20 1.375 μm 27.5 μm 10x 0.25 1.10 μm 17.6 μm 10x 0.45 0.61 μm 5.43 μm 20x 0.40 0.69 μm 6.87 μm 20x 0.75 0.37 μm 1.95 μm 40x 0.65 0.42 μm 2.60 μm 40x 1.40 0.20 μm 0.56 μm 63x 0.75 0.37 μm 1.95 μm 63x 1.30 0.25 μm 0.65 μm 100x 1.25 0.22 μm 0.70 μm 100x 1.40 0.20 μm 0.56 μm Carl Zeiss Microscopy 1/28/2019 11 Aspects of Resolution Enhancing Axial Resolution REQUIREMENTS: • Collect images with Z step sizes smaller than the target resolution - Motorized focus drive • Imaging method that results in a clearer “slice” of the source signal - Optical sectioning technique Carl Zeiss Microscopy 1/28/2019 12 Outline of Discussion High Resolution Optical Sectioning 1 Basic Aspects of Resolution in X, Y, and Z 2 Modalities for Enhancing Axial Resolution 3 Advanced Approaches for Exceeding the Resolution Limit 4 Summary of Techniques 5 Questions Carl Zeiss Microscopy 1/28/2019 13 Optical Sectioning Techniques Hierarchy of Common Approaches Sectioning Methods Optics Optics & Mathematics Mathematics Light Sheet Confocal Total Internal Structured 3D Multi-Photon Microscopy Microscopy Reflection Illumination Deconvolution Carl Zeiss Microscopy 1/28/2019 14 Optical Sectioning Confocal Microscopy Sectioning Methods Optics Optics & Mathematics Mathematics Light Sheet Confocal Total Internal Structured 3D Multi-Photon Microscopy Microscopy Reflection Illumination Deconvolution Carl Zeiss Microscopy 1/28/2019 15 Confocal Microscopy Rejection of Out-of-Focus Signal Excitation Emission Widefield Widefield ZEISS ZEISS Plan-NEOFLUAR Plan-NEOFLUAR 40x /1,3 Oil 40x /1,3 Oil Excitation Emission Confocal Confocal ZEISS ZEISS Plan-NEOFLUAR Plan-NEOFLUAR 40x /1,3 Oil 40x /1,3 Oil Carl Zeiss Microscopy 1/28/2019 16 Confocal Microscopy Rejection of Out-of-Focus Signal • Laser excitation and sample Detector emission separated by dichroic Laser Sample / Focal Plane Carl Zeiss Microscopy 1/28/2019 17 Confocal Microscopy Rejection of Out-of-Focus Signal • Laser excitation and sample Detector emission separated by dichroic Pinhole Confocal Plane • The pinhole(s) prevent detection of out-of-focus signal - Diaphragm situated in conjugate focal plane Laser Sample / Focal Plane Carl Zeiss Microscopy 1/28/2019 18 Confocal Microscopy Rejection of Out-of-Focus Signal • Laser excitation and sample Detector emission separated by dichroic Pinhole Confocal Plane • The pinhole(s) prevent detection of out-of-focus signal - Diaphragm situated in conjugate focal plane Laser • Thickness of resulting optical section is influenced by: - Numerical aperture of lens - Wavelength of excitation light - The shape, spacing, and diameter of the pinhole Sample / Focal Plane Carl Zeiss Microscopy 1/28/2019 19 Confocal Microscopy Methods for Scanning • Increased scanning speeds are possible by multipoint approaches - Such approaches sacrifice pinhole versatility at emission side and thus have less control over the optical section thickness Point Scanning Confocal Line Scanning Confocal Spinning Disk Confocal (single point at a time) (single line or slit at a time) (~1000 points at a time) Carl Zeiss Microscopy 1/28/2019 20 Point-Scanning Confocal Basic Principles • A diffraction-limited laser spot is moved across the sample via two independent scanning mirrors - The resulting image is generated a single point at a time Carl Zeiss Microscopy 1/28/2019 21 Point-Scanning Confocal Common/Unique Applications WHAT ARE THE USES? • Easy-to-implement simultaneous Cells detector layout enables true spectral imaging - Separation of closely-overlapping fluorophores, identification of autofluorescent populations Morphology 32-channel spectral readout of giant unilamellar Visualization of gut immune cell populations vesicle (GUV) labelled with laurdan Hugues Lelouard et al (Aix Marseille Univ, France) Carl Zeiss Microscopy 1/28/2019 22 Point-Scanning Confocal Common/Unique Applications WHAT ARE THE USES? • Control of pointwise illumination with nanosecond-scale photon readout permits measurement/modeling of molecular dynamics - FRAP mobility (parameters of diffusion, viscosity, size), transport kinetics - FCS diffusivity, concentration, transport and binding kinetics - RICS spatial maps of diffusivity, concentration(s) - N&B concentration, oligomerization Detailed characterization of any aqueous compartment or environment via fluorescence! FCS RICS Carl Zeiss Microscopy 1/28/2019 23 Point-Scanning Confocal Typical System Footprint – ZEISS LSM 880 LSM 880 scanhead; 34-channel (GaAsP) Transmitted light detector (“T-PMT”) Laser lines: 405, 458, 488, 514, 561, 633 nm Objectives: 10x/0.3 40x/1.3 oil 25x/0.8 oil/W 63x/1.4 oil 40x/1.2 W 100x/1.46 oil Motorized XY stage + Z-piezo insert Observer inverted microscope Carl Zeiss Microscopy 1/28/2019 24 Point-Scanning Confocal Practical Perspective ADVANTAGES: • Nearly ubiquitous 3D modality • Easy to combine multiple channels in parallel (spectral imaging) • Precise pixel photomanipulation possible • Works with reflected mode imaging (non-fluorescent structures) FITC electrospun fiber scaffold (Xie Lab, Univ. Nebraska Med.) DISADVANTAGES: • Relatively slow point-by-point acquisition • High laser powers may cause photobleaching or photodamage • Photocathode-based detectors are less sensitive than cameras Fluorescent flake on paper (reflection) Carl Zeiss Microscopy 1/28/2019 25 Spinning Disk Confocal Basic Principles • A single rotating disk containing hundreds of pinholes scans laser light across the sample - Rotation speed is adjustable (~1500 – 5000 RPM) • Paul Nipkow patented concept in 1884, later became basis for mechanical television in 1920s Carl Zeiss Microscopy 1/28/2019 26 Spinning Disk Confocal Basic Principles • Pinholes are arranged along slightly curved line (Archimedean spiral) • Over a set exposure time, these lines assemble into one full scan of the field of view - 12 scans per complete rotation, 50 μm pinhole diameter, 250 μm apart • To evenly scan, rotation speed and exposure time are synchronized Carl Zeiss Microscopy 1/28/2019 27 Spinning Disk Confocal Basic Principles • Pinholes illuminated by laser (via focusing of microlens array) - Projected onto sample • Emission collected through objective; passes pinhole array - Only in-focus signals • Beamsplitter passes fluorescence to area detector (e.g. – CCD) - High-speed confocal Carl Zeiss Microscopy 1/28/2019 28 Spinning Disk Confocal Common/Unique Applications Drosophila embryo (projection) Zebrafish red blood cells WHAT ARE THE USES? • Projects requiring high-throughput 3D imaging, up to 2 simultaneous channels • Increasingly central to screening studies (e.g.
Details
-
File Typepdf
-
Upload Time-
-
Content LanguagesEnglish
-
Upload UserAnonymous/Not logged-in
-
File Pages85 Page
-
File Size-