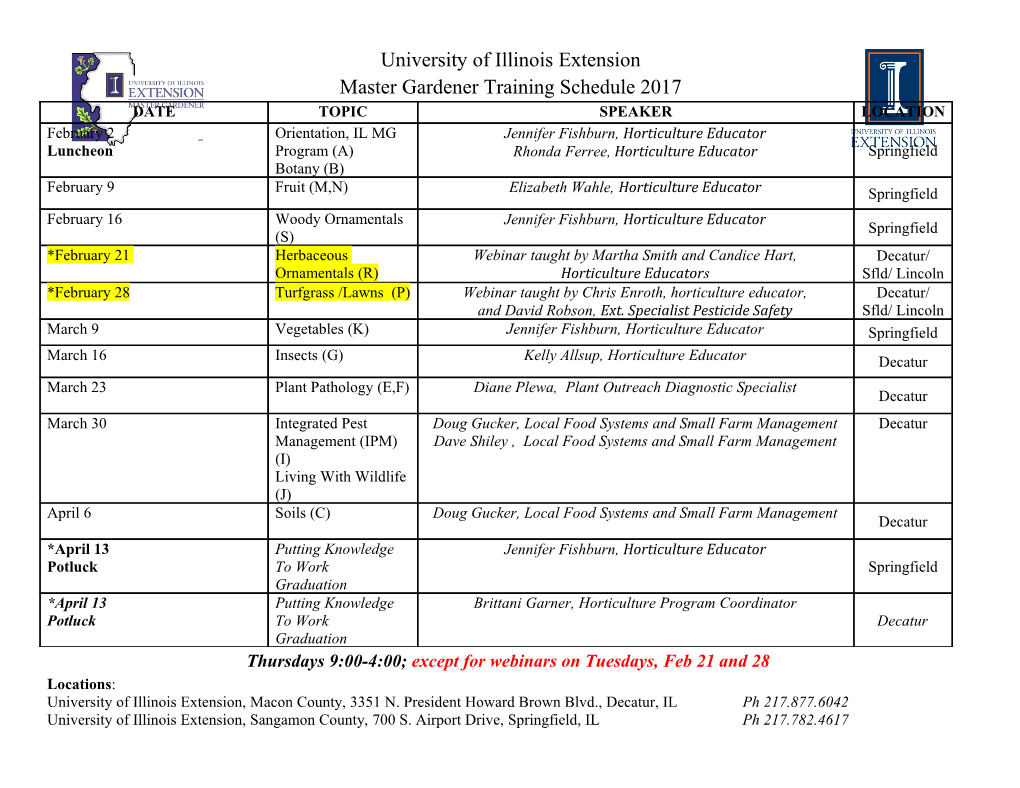
Review Building bridges within the bacterial chromosome 1,2 2 Dan Song and Joseph J. Loparo 1 Harvard Biophysics Program, Harvard Medical School, Boston, MA, USA 2 Department of Biological Chemistry and Molecular Pharmacology, Harvard Medical School, Boston, MA, USA All organisms must dramatically compact their genomes called macrodomains (Box 1) with a size of approximately to accommodate DNA within the cell. Bacteria use a set 1 Mb [2,3]. of DNA-binding proteins with low sequence specificity For the past two decades, studies on bacterial chromo- called nucleoid-associated proteins (NAPs) to assist in some condensation and organization have focused on NAPs chromosome condensation and organization. By bend- that can be classified as DNA benders or DNA bridgers ing or bridging DNA, NAPs also facilitate chromosome (Figure 1). Examples of well-studied DNA benders include segregation and regulate gene expression. Over the past HU (a histone-like protein from Escherichia coli strain U93) decade, emerging single-molecule and chromosome and integration host factor (IHF). These proteins can non- conformation capture techniques have investigated specifically bind to DNA by inserting two arms into the minor the molecular mechanisms by which NAPs remodel groove, inducing curvature of more than 908 [4]. DNA and organize the bacterial chromosome. In this review bridgers form dynamic connections between DNA duplexes we describe how such approaches reveal the biochemi- resulting in large DNA loops that can span several kilobases. cal mechanisms of three NAPs that are believed to Besides condensing chromosomes, DNA bridgers also func- facilitate DNA bridging: histone-like nucleoid structuring tion in chromosome segregation and transcription silencing. protein (H-NS), ParB, and structural maintenance of In this review we focus on how single-molecule and chromo- chromosomes (SMC). These three proteins form quali- some conformation capture techniques (Box 2 and Table 1) tatively different DNA bridges, leading to varied effects are contributing to our understanding of how bacteria orga- on transcription and chromosome segregation. nize and compact their chromosomes. As a case study, we examine three NAPs – H-NS, ParB, and SMC – believed to be Shaping the bacterial chromosome DNA bridgers. We describe how these proteins form quali- Both eukaryotic and prokaryotic organisms must dramati- tatively different protein–DNA bridges and speculate on cally condense their genomic DNA to package it into cells how these differences might be important in determining that are only about one thousandth their length. Simulta- their unique biological functions. For additional discussion of neously, DNA must remain accessible for essential pro- NAPs and their functions, see the reviews in [5,6]. cesses like DNA replication, repair, transcription, and chromosome segregation. In eukaryotes, genomic DNA is H-NS wrapped around histone proteins to form nucleosomes The enterobacterial H-NS is a small and abundant protein that are further organized into higher-order structures. that is involved in both chromosome organization and gene Bacteria lack histones but have evolved other mechanisms regulation [7,8]. Although H-NS lacks a consensus DNA- to condense and organize their chromosomes. These binding sequence, it binds with high affinity to AT-rich factors include DNA supercoiling, macromolecular crowd- DNA sequences that display sequence-induced curvature ing, the highly conserved condensin complexes comprising [9–11]. Bacterial promoters commonly comprise such structural maintenance of chromosomes (SMC) proteins motifs and H-NS specifically binds to these sites, influenc- and their subunits, and the small NAPs that are unique ing the regulation of a wide variety of genes [12,13]. Exam- to bacteria. SMCs and NAPs play an important role in ples of H-NS-regulated genes include its own gene, hns not only maintaining genome compaction but also dynam- [14], rrnB, which is responsible for ribosomal RNA synthe- ically reorganizing the genome to make it accessible for sis [15], proU, which controls an osmotically inducible gene expression. By specifically and nonspecifically bind- transport system [16], and horizontally acquired virulence ing to the genome, NAPs appear to contribute to the genes such as virF [17]. Mutations in hns typically cause formation of isolated topological regions known as micro- an increase in transcription, indicating that H-NS is pre- domains (Box 1) with an average size of 10 kb [1]. These dominantly a gene silencer [18]. microdomains are further organized into larger regions How H-NS negatively regulates transcription remains controversial. For some genes, such as the Shigella virB, Corresponding author: Loparo, J.J. ([email protected]). Keywords: single molecule; chromosome organization; nucleoid-associated protein; H-NS binding sites overlap the promoter region; in these structural maintenance of chromosomes; DNA bridging. cases, H-NS is likely to occlude RNA polymerase binding 0168-9525/ [19]. For others, such as the E. coli and Salmonella typhi- ß 2015 Elsevier Ltd. All rights reserved. http://dx.doi.org/10.1016/j.tig.2015.01.003 murium proU operons, H-NS influences transcription 164 Trends in Genetics, March 2015, Vol. 31, No. 3 Review Trends in Genetics March 2015, Vol. 31, No. 3 Box 1. Domains within the bacterial chromosome The bacterial chromosome is organized into domains of various determinant of boundaries was the presence of highly expressed genes lengths. On the smallest level, chromosomal DNA is divided into where the DNA is kept free of plectonemic loops by active transcription microdomains with an average size of 10 kb [1]. These domains are [i.e., mechanism (ii) above]. negatively supercoiled and form plectonemic loops that are topolo- On the significantly longer length scale of micrometers, the bacterial gically insulated from each other [1,91,92]. In the popular bottlebrush chromosome is further organized into macrodomains. Experimental model of the bacterial chromosome, microdomains form the bristles efforts in Escherichia coli that measured chromosome dynamics by site- of the brush that extend out from a dense DNA core [93]. Micro- specific labeling [2,94] and recombination frequency along the chromo- domains prevent relaxation of the entire chromosome on single- some [3] identified the Ori, Ter, Left, and Right macrodomains along with strand nicking; such unraveling would have disastrous consequences two unstructured regions. Each macrodomain is approximately 1 Mb. given that plectonemic loops substantially compact the chromosome. The molecular origins of macrodomain organization remain unclear. The formation of insulated topological domains requires boundary Potential domainins such as MatP and SeqA have been identified based regions that are kept free of plectonemic loops. This can be on both in vivo DNA-binding profiles using ChIP [95,96] and genome accomplished by two mechanisms: (i) preventing rotation of the DNA conformation capture [40]. Unlike NAPs, MatP and SeqA do not have at the domain boundaries (Figure IA); or (ii) keeping the DNA within any influence on gene expression and exhibit high sequence specificity, these regions in an unwound state (Figure IB). NAPs, especially those binding either exclusively inside or outside the Ter macrodomain. MatP involved in DNA bridging, have long been assumed to contribute to recognizes 23 specific DNA-binding sites known as matS sites within domain formation (so called domainins) by acting through the first the Ter region [95]. Structural studies demonstrate that MatP tetramers mechanism. Growing evidence, however, suggests that NAPs may can bridge distant matS sites to organize the Ter domain by DNA instead act by condensing DNA within a microdomain. Chromosome condensation [97]. SeqA, by contrast, can sequester newly replicated conformation capture coupled with deep sequencing (see Table 1 in origins by binding to hemimethylated sites [98]. Thus, it was proposed main text) in Caulobacter crescentus showed that deletion of HU and that SeqA can organize the Ori domain by properly orientating the SMC only weakly affected local domain boundaries [78]. The strongest chromosome during replication [99]. (A) (B) (−) Key: RNA Pol Transcript Domain 1 (−) (+) Domain 1 Domain 2 Domain 2 (−) Transcripon direcon TRENDS in Genetics Figure I. Models of DNA topological domain formation. (A) By bridging DNA segments, nucleoid-associated proteins (NAPs) (purple circles) can prevent the rotation of negatively supercoiled DNA at domain boundaries. (B) During transcription, positive and negative supercoils are generated ahead and behind RNA polymerase. By keeping the intervening DNA in an unwound state, transcription acts to keep the domains separated. Note that the DNA and proteins are not drawn to scale. despite binding downstream of the promoter [16,20]. The molecular mechanism by which H-NS bridges DNA Some clues to the functional role of H-NS in transcription is closely related to its structure. H-NS is a 15.6-kDa can be gained by understanding how H-NS interacts protein with an N-terminal oligomerization domain and with DNA. Early in vitro studies demonstrated that a C-terminal DNA-binding domain connected by a flexible purified H-NS can condense DNA [21] and overexpression linker (Figure 2A) [27,28]. The N-terminal domain of H-NS of H-NS in E. coli was found to compact the nucleoid is made of a short coiled-coil motif that can interact in an and cause cell death [22]. Subsequent
Details
-
File Typepdf
-
Upload Time-
-
Content LanguagesEnglish
-
Upload UserAnonymous/Not logged-in
-
File Pages10 Page
-
File Size-