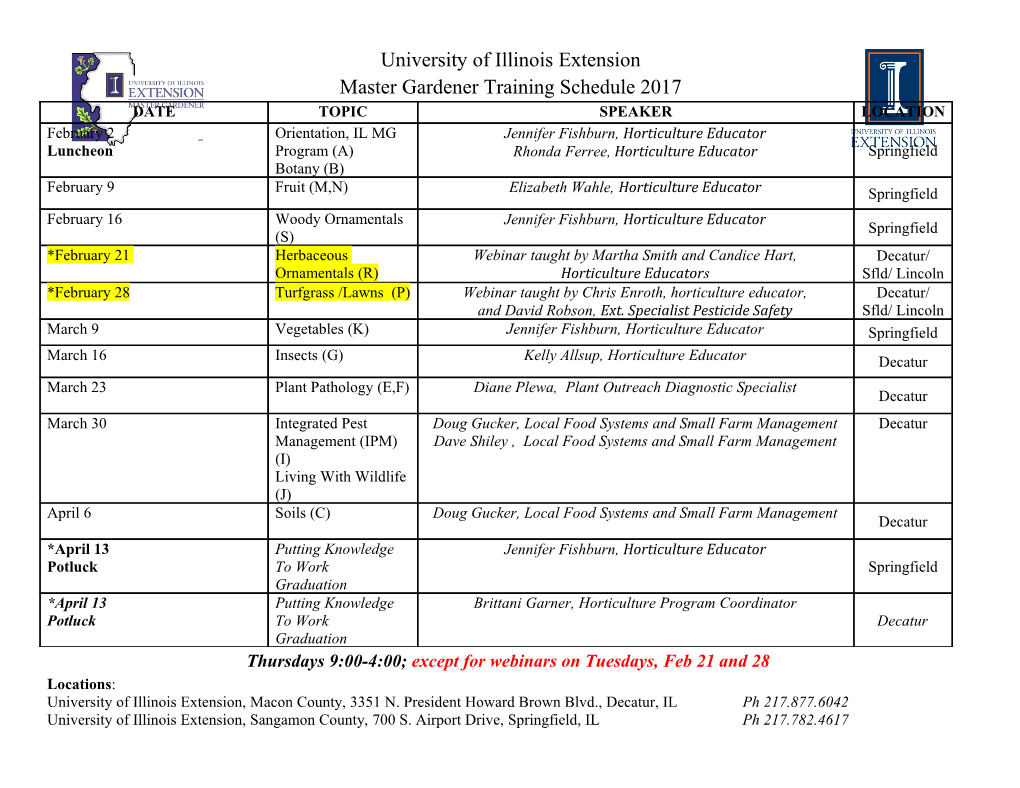
Cover Page The handle http://hdl.handle.net/1887/44483 holds various files of this Leiden University dissertation Author: Otten, Gilles Title: Suppressing a sea of starlight : enabling technology for the direct imaging of exoplanets Issue Date: 2016-11-29 1| Introduction Mankind has often philosophized about the existence of planets around other stars. It has been recorded as early as 300 BC when Epicurus argued, in a letter to his disciple Herodotus, that innumerous worlds must exist, both similar and dissimilar to ours (Laertius, 300). Later in the 17th century, Christiaan Huygens wrote in his philosophical treatise Cosmotheoros on the existence of planets around other stars, as well as seas, mountains, plants and animals on those planets1. Using our modern view we can tackle this problem more scientifically. We can formulate more structured questions such as; how do planets form?; is our solar system normal or special?; how unique is our earth? and finally, the ultimate question; is there life elsewhere in the universe? To answer these questions we need to discover and study planets around other stars. Planets around other stars (extrasolar/exoplanets) were first discovered in 1992 when Wol- szczan and Frail discovered two low mass exoplanets around the pulsar PSR1257+12 based on periodic variations of the pulsar’s pulse arrival times (Wolszczan and Frail, 1992). This long- expected confirmation proved that planets existed around other (albeit dead) stars. The huntwas on to find a planet around a solar-like star. In 1995, Mayor and Queloz managed to detect radial velocity variations of the solar-type star 51 Pegasi that implied the presence of a planet of at least 0.47 Jupiter masses (MJup)(Mayor and Queloz, 1995), where there is a lower limit depending on the inclination of the orbit. In the following years more planets were discovered using the radial velocity (RV) technique (e.g. Marcy and Butler, 1996; Butler and Marcy, 1996) and it was evident that the first detected objects were mainly roughly Jupiter-mass planets at unexpectedly small Mercury-like orbits. The big question was how planets could be present at these locations. Quickly the realization came that the prob- ability of such an object passing directly in front of its star as seen from Earth was about 10%. This transit would be seen as a small periodic decrease in the amount of light received fromthe parent star as the planet physically blocked part of the starlight. The first planet detected using this transit method was HD 209458 b by Charbonneau et al. (2000); Henry et al. (2000), which was originally discovered with the radial velocity technique. This method allows for a relatively easy way of searching for exoplanets by monitoring thebright- ness of thousands of stars simultaneously. Within several years it has quickly become the most prolific planet detection method, especially with dedicated projects such as Kepler andSuper- WASP. The detections of exoplanets through both RV and transit studies have raised questions on planet formation and migration (Udry et al., 2007), and has given us constraints on the occur- rence of different types of planets (down to Earth radii) at different orbits around stars(Fressin et al., 2013). Two other important techniques to discover exoplanets are microlensing (Gaudi, 2012) and astrometry. Astrometry is related to the RV method as it also uses the motion of the parent star to infer the presence of companions. The reflex motion of the star caused by the presence ofacom- 1http://www.staff.science.uu.nl/˜gent0113/huygens/huygens_ct_en.htm 2 Introduction panion also has a transverse component that can be measured by accurately recording the position of the star in the plane of the sky. Previously, this technique was used to support several claimed detections of extrasolar planets. Most notoriously, the detection of two sub-Jupiter-mass planets around Barnard’s star was presented by van de Kamp (1982) but later studies showed that these signals were spurious as planets have been excluded down to several Neptune masses (M. Kürster et al., 2003). A candidate was finally found with astrometry by Muterspaugh et al. (2010) orbit- ing in a binary system. This planet still has to be confirmed and it is unknown around whichof the binary stars it orbits, thereby its properties are still ambiguous. A previously known exoplanet Gl876 b has also been confirmed with the Hubble Space Telescope (HST) through astrometry (Benedict et al., 2002). While this technique still has to discover its first official confirmed planet it is expected that the Gaia satellite, with its high astrometric precision, will detect thousands of Jupiter-mass objects within its lifetime (Perryman et al., 2014). Microlensing uses the effect that a massive object acts as a lens and distorts space-time around it. This lensing effect increases the amount of light that is received from a star behind thefore- ground star + planet combination. This brightening follows from Einstein’s theory of general rela- tivity and the magnification factor was consequently derived by Einstein (1936). Deviations from a simple lens can indicate the presence of a companion. The paper by Paczynski (1986) predicted that dark matter candidates could be found using this method by observing millions of stars for several years. Later, Mao and Paczynski (1991) wrote that exoplanets located at approximately the Einstein radius away from the star would be detectable in a similarly sized sample of stars. The first detection using the microlensing method was the 3 MJup planet OGLE 2003-BLG-235 b/MOA 2003-BLG-53 b by Bond et al. (2004). The current discoveries with the major detection methods, with known mass and separation, are shown in Fig. 1.1. This plot includes discoveries made by the direct imaging technique thatwill be described in Section 1.1. Each of the methods has their advantages and disadvantages (see Table 1.1). Pulsar timing has led to the discovery of planets in a handful of cases, as the planets need to survive stellar death or otherwise form around a dead star. Microlensing candidates can only be studied during a single statistically unlikely event. Astrometry is expected to become routine with the astrometric performance of Gaia. The radial velocity and transit methods are currently the most prolific methods but are mainly sensitive to planets close to the star. The direct imaging method described in this paper overcomes many of these biases. The previously mentioned detection methods are indirect ways of determining properties of the exoplanets such as period, radius and mass. Periods are easy to determine and can be translated into orbital radii with an estimate of the mass of the star. The radius is determined by measuring the amount of blocked light during a transit. A lower limit to the mass can be derived through radial velocity measurements. When combined with the radius of transiting planets this yields the density and therefore can give an indication of the bulk composition of the planet. Several methods are also sensitive to the properties of the planetary atmosphere, like wind speed (Snellen et al., 2010) and chemical abundances (Seager and Deming, 2010). For instance, the transit method can detect atmospheric features imprinted on the stellar light (Charbonneau et al., 2002) when the planet passes directly in front of its star. The presence of a spectral feature will appear as an apparent increase in the amount of blocked light and therefore derived radius of the planet. In the case of relatively hot planets, the thermal emission of the day-side of the planet can even be recorded during the secondary eclipse when the planet hides behind the star. The secondary eclipse probes the thermal radiation of the planet at the height in the atmosphere where it becomes 1.1 The direct imaging of exoplanets 3 Figure 1.1: Mass versus separation plot for currently known extrasolar planets. This plot also includes some candidates that span the transition between planets and brown dwarfs. The official IAU definition of a planet has a cut-offat13 MJup. Source: www.exoplanet.eu (October 2015) and known solar-system values. optically thin. Different wavelengths therefore probe different heights in the atmosphere andcan be used to constrain atmospheric temperature/pressure profiles. Using the radial velocity method at high spectral resolution (R ∼ 100 000) it is also possible to probe the atmosphere by stacking the resolved spectral features of the planet using an atmospheric template (Snellen et al., 2010). The rotation rate of the known planet Beta Pictoris b was also determined with this technique (Snellen et al., 2014). 1.1 The direct imaging of exoplanets The previously mentioned detection methods mostly depend on information derived fromthe light of the star and are therefore indirect ways of probing exoplanets. With direct imaging, the light of the planet itself is detected spatially resolved from the star, yielding a direct measurement of the thermal and/or reflected light of a planet. With enough spectral resolution and signal- to-noise, determination of atmospheric composition is possible. This makes it a very powerful technique for directly studying the atmospheres of planets. Furthermore, direct imaging does not require a full orbit of a planet around its star to confirm it is real. The technique of direct imaging is biased towards planets at larger separations from thestar (5-1000 AU). This complements the other methods that are more sensitive to planets that areclose
Details
-
File Typepdf
-
Upload Time-
-
Content LanguagesEnglish
-
Upload UserAnonymous/Not logged-in
-
File Pages41 Page
-
File Size-