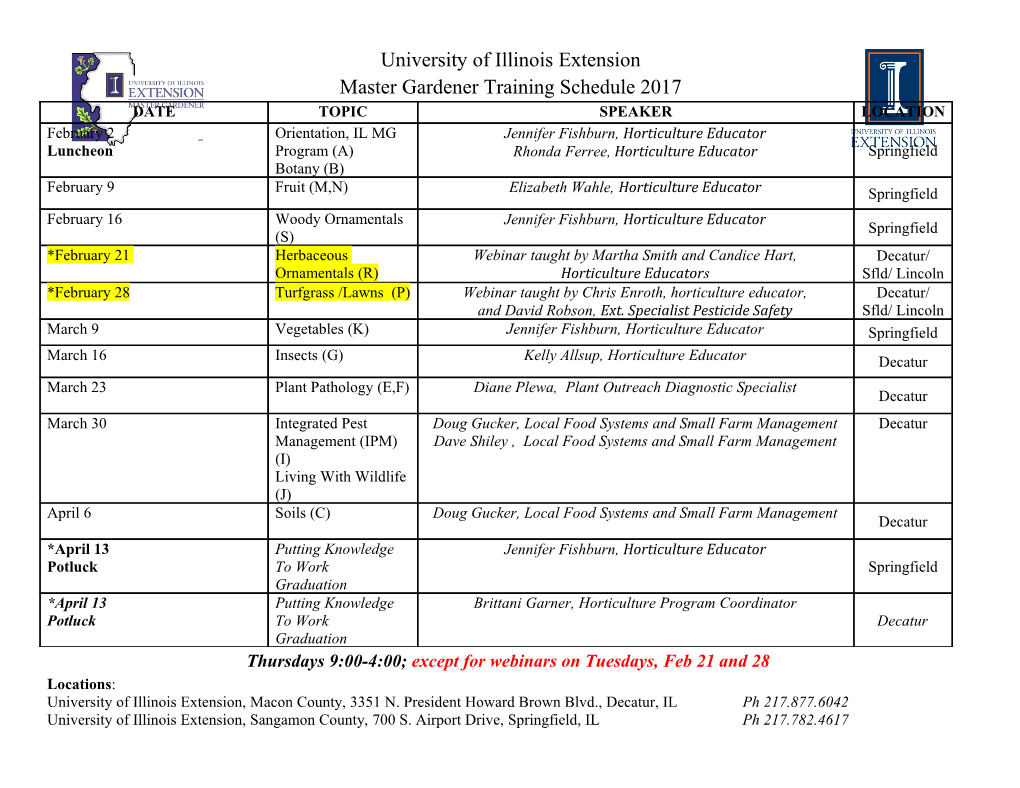
Plant Molecular Biology 26: 1557-1577, 1994. © 1994 Kluwer Academic Publishers. Printed in Belgium. 1557 Current advances in abscisic acid action and signalling J6r6me Giraudat*, Francois Parcy, Nathalie Bertauche, Frangoise Gosti, Jeffrey Leung, Peter-Christian Morris, Michelle Bouvier-Durand and Nicole Vartanian Institut des Sciences VOgOtales, Centre National de la Recherche Scientifique UPR 40, 91198 Gif-sur- Yvette Cedex, France (* author for correspondence) Received and accepted 21 June 1994 Key words: abscisic acid, gene regulation, mutants, seeds, stomata, stress Abstract Abscisic acid (ABA) participates in the control of diverse physiological processes. The characterization of deficient mutants has clarified the ABA biosynthetic pathway in higher plants. Deficient mutants also lead to a revaluation of the extent of ABA action during seed development and in the response of vegetative tissues to environmental stress. Although ABA receptor(s) have not yet been identified, considerable progress has been recently made in the characterization of more downstream elements of the ABA regulatory network. ABA controls stomatal aperture by rapidly regulating identified ion trans- porters in guard cells, and the details of the underlying signalling pathways start to emerge. ABA actions in other cell types involve modifications of gene expression. The promoter analysis of ABA-responsive genes has revealed a diversity of cis-acting elements and a few associated trans-acting factors have been isolated. Finally, characterization of mutants defective in ABA responsiveness, and molecular cloning of the corresponding loci, has proven to be a powerful approach to dissect the molecular nature of ABA signalling cascades. Introduction the underlying regulatory pathways. The first part of this article is thus devoted to an update of Abscisic acid (ABA) is a naturally occurring plant certain aspects of ABA physiology and in par- hormone (or growth regulator) that was identified ticular of the information derived from the char- in the 1960s (see [2] for a review of the early acterization of ABA-biosynthetic mutants. This studies on ABA). ABA is probably present in all brief overview is only meant to provide the nec- higher plants, and has been implicated in the con- essary background to studies on ABA signalling; trol of a wide range of essential physiological pro- further details on ABA functions can be found in cesses including seed development and plant ad- several books and reviews [1, 18, 64, 166, 175]. aptation to environmental stress. In the second part, we then analyse more exten- The present review emphasizes the recent ad- sively the respective contribution of various ex- vances made in elucidating the ABA signal trans- perimental approaches to our present knowledge duction pathways. Identification of plant re- of the molecular cascades mediating ABA effects sponses that are regulated by ABA in vivo is a at the cellular level. prerequisite to studies aiming at characterizing [321] 1558 Recent advances from the analysis of ABA- Mevalonic acid deficient mutants I V Famesyl pyrophosphate (C 15) As for many other biologically active substances, I vp2 the possible roles of ABA in plants were initially I vp5 investigated by monitoring endogenous ABA [Carotenoids] I vp7 vp9 contents and by analysing the effects of exog- enously applied ABA or biosynthetic inhibitors Zeaxanthin (C40) (reviewed in [166]). Mutants deficient in ABA [ aba synthesis provide a means to more directly assess Antheraxanthin the roles of ABA under physiological conditions. aba all-trans-Violaxanthin As will be described below, such mutants lead us to a reevaluation of the extent of ABA action 9'-cis-Neoxanthin (C40) during seed development and in the response of vegetative tissues to various stress conditions. XantSoxin (C15) Deficient mutants have also contributed exten- sively to the clarification of the biosynthetic path- ABA-aldehyde flacca ways of ABA. A"~ ~ sitiens ABA-alcohol droopy .~ abal "~ "~ nar2a The ABA biosynthetic pathway in plants ~"~ ABA Mutants that are deficient in ABA have been iso- O~COOH lated in a variety of species (see Table 1). Among the best characterized are the maize viviparous Fig. I. Simplified pathway of ABA biosynthesis in higher ( vp) [ 114, 134], tomato flacca, sitiens and notabilis plants. The metabolic blocks in various ABA-deficient mu- [153, 156], Arabidopsis aba [79], potato droopy tants are indicated. Adapted from [155, 167, 175, 176]. [131], pea wilty [168] and Nicotianaplumbagini- folia abal [ 123, 139] mutants. These mutants dis- play various abnormalities that, as expected for ing zeaxanthin to antheraxanthin and most likely mutants deficient in the hormone, can be restored in the subsequent one leading to violaxanthin [26, to wild type by exogenous supply of ABA. 137]. The tomato flacca and sitiens [ 156], potato ABA is a sesquiterpenoid with mevalonic acid droopy [27 ] and N. plumbaginifolia abal [ 123, 139] as its precursor. While phytopathogenic fungi mutants are all blocked in the final step(s) of ABA synthesize ABA by a direct pathway (also known biosynthesis, oxidation of ABA aldehyde to ABA. as the C15 pathway) from farnesyl pyrophosphate, This step might be catalyzed by an enzyme that numerous distinct lines of evidence now support requires a molybdenum cofactor, as suggested in that higher plants rather synthesize ABA by an particular by the barley nar2a mutant [ 165]. De- indirect (or C40) pathway from xanthophylls (see fects in such a cofactor might conceivably affect [ 155, 167, 175, 176] for detailed reviews). In par- additional reactions unrelated to ABA biosynthe- ticular, the biosynthetic defects identified in sis and thus possibly explain the pleiotropic effects ABA-deficient mutants are congruent with the in- of the barley nar2a [165], tomato flacca [152] direct pathway schematically depicted in Fig. 1. and N. plumbaginifolia abal [ 123, 139] mutations. The maize vp2, vp5, vp7 and vp9 mutants are In addition, the existence of a minor shunt path- blocked in the early stages of carotenoid biosyn- way that involves reduction of ABA aldehyde to thesis [108, 114]. The Arabidopsis aba mutants ABA alcohol and oxidation of ABA alcohol to are impaired in the epoxidation reaction convert- ABA (most likely via a cytochrome P-450 mono- [3221 1559 oxygenase) was uncovered by the tomato flacca Roles of ABA during seed development and sitiens [136] and other mutations (reviewed in [ 136, 155, 176]). Other ABA-biosynthetic mu- ABA has been proposed to be an essential regu- tations have not yet been unambiguously related lator of various processes occurring during to a particular step of the pathway (discussed in roughly the last two thirds of seed development, [155]). i.e. during the 'maturation' and 'post-abscission' As illustrated below for some ABA-response phases in the nomenclature proposed by Galau et mutants, techniques are now available to clone a al. [38]. In a number of mono- and di- gene simply on the basis of its associated mutant cotyledonous species, endogenous ABA content phenotypes. The available ABA-biosynthetic mu- has indeed been shown to peak during this period tants might thus lead in the near future to the before returning to low levels in the dry seed (for identification of genes encoding various enzymes reviews see [6, 132]). involved in ABA biosynthesis. These would be When removed from the ovule after the end of valuable tools to improve our understanding of pattern formation, most embryos display the abil- the regulation of ABA biosynthesis but also to ity to germinate precociously on culture medium. better identify which cells synthesize ABA. Such precocious germination could be prevented Table 1. Characteristics of the various ABA mutants. Additional details on the mutant phenotypes can be found in the text. The loci that have been cloned are in bold, and the potential function of the encoded protein is indicated. Species Name Defect References ABA-deficient mutants Z. mays vp2 carotenoid biosynthesis [ 108, 114, 134] vp5 carotenoid biosynthesis [108, 114, 134] vp7 carotenoid biosynthesis [108, 114, 134] vp9 carotenoid biosynthesis [ 108, 114, 134] vp8 ? [108, 114, 134] A. thaliana aba epoxidation of xanthophylls [26, 79, 137] L. esculentum notabilis ? [ 153] flacca oxidation of ABA aldehyde to ABA [153, 156] sitiens oxidation of ABA aldehyde to ABA [ 153, 156] S. phureja droopy oxidation of ABA aldehyde to ABA [27, 131] P. sativum wilty ? [27, 1681 N. plumbaginifolia abal/ckrl oxidation of ABA aldehyde to ABA [ 123, 139] H. vulgare nar2a oxidation of ABA aldehyde to ABA [ 165] (deficient in molybdenum cofactor) ABA-insensitive mutants A. thaliana abil ABA responsiveness [8O] (Ca2 +-modulated protein phosphatase) [86, 1061 abi2 ABA responsiveness [801 abi3 ABA responsiveness, seed-specific [80, 112, 119] (transcription activator) [451 abi4 ABA responsiveness (seed-specific?) [341 abi5 ABA responsiveness (seed-specific?) [34] Z. mays vpl ABA responsiveness, seed-specific [100, 134, 135] (transcription activator) [102] H. vulgare cool ABA sensitivity in guard cells [133] [323] 1560 in many cases by adding ABA to the culture me- marker mRNAs both in vivo [68] and under vari- dium, thereby suggesting that the increased seed ous culture conditions [69], Hughes and Galau ABA levels play a similar role in vivo (for reviews have identified several classes of coordinately ex- see [6, 132]). The various nonallelic ABA- pressed mRNAs. Their expression patterns can biosynthetic viviparous mutants
Details
-
File Typepdf
-
Upload Time-
-
Content LanguagesEnglish
-
Upload UserAnonymous/Not logged-in
-
File Pages21 Page
-
File Size-