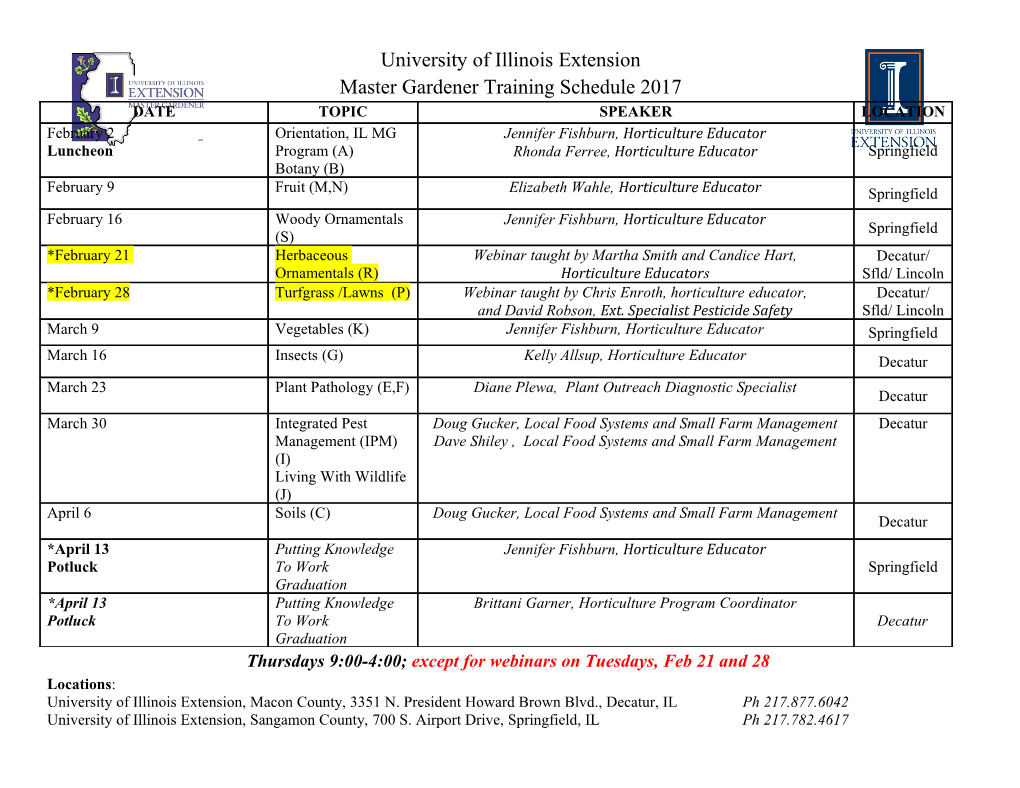
energies Article Empirical and Numerical Analysis of Conduction and Induction Charging of Droplets in a Three-Electrode System Adam Pelesz * and Tomasz Czapka * Department of Electrical Engineering Fundamentals, Faculty of Electrical Engineering, Wrocław University of Science and Technology, 50-370 Wrocław, Poland * Correspondence: [email protected] (A.P.); [email protected] (T.C.) Received: 20 November 2019; Accepted: 16 January 2020; Published: 18 January 2020 Abstract: The charging process of water droplets in different electrification systems using empirical and numerical analysis is described. A simplified model based on capacitance distribution is proposed. The results of the experimental studies and numerical calculations enable comparison of the induction and conduction charging methods in terms of the droplet charging level, described by the Q/m parameter. Research findings indicate that the conduction method in a 3-electrode system is the most effective among all the considered methods. It has been also shown that the application of a 2-electrode system under certain conditions provides greater Q/m parameter values in comparison to the system with three electrodes using the induction method. The research results can be used to develop charging systems (e.g., nozzles) that produce streams with electrically charged liquid particles. Keywords: electroaerosol; electrification of droplets; induction electrification 1. Introduction Charging of aerosol particles is widely used in many technological processes [1–4]. Among the most common applications, one can mention agriculture (e.g., pesticide application and artificial pollination) [5–9], medicine (e.g., inhalation medications) [10,11], industry (e.g., painting, xerography, and food industries) [12–15], and others (e.g., thin film deposition, cosmetology, insecticide spraying) [16–18]. The use of electroaerosols improves the efficiency of a given process, for example providing more uniform object coverage and increasing the coating efficiency [19–21]. In general, there are several methods of aerosol charging, such as induction, conduction, corona discharge, postdispersion, and triboelectrification methods [2,12,13,22–27]. Induction and conduction charging methods are commonly used for conducting liquids. Furthermore, both methods can be implemented in two- or three-electrode systems. All mentioned methods, which require an external high voltage source, are schematically shown in Figure1. It should be noted that both conduction and induction methods are based on the principle of electrostatic induction. The key difference between them is the electric potential of the sprayed liquid. In the case of the induction method, the sprayed liquid is charged while being on ground potential. On the contrary, in the case of the conduction method, the liquid is charged at high potential (negative or positive). For a 2-electrode system, the induction and conduction methods are equivalent in terms of electric field distribution (i.e., if the influence of other surrounding objects, which may act as additional electrodes, are omitted). Moreover, in the case of a 3-electrode system, despite the same geometry (i.e., electrode configuration), there are some important differences, including in the electric field distribution, energy conversion, and the space charge effect. A more detailed description of the mentioned differences can be found in [25]. Energies 2020, 13, 469; doi:10.3390/en13020469 www.mdpi.com/journal/energies Energies 2020, 13, x FOR PEER REVIEW 2 of 15 field distribution, energy conversion, and the space charge effect. A more detailed description of the Energies 2020, 13, 469 2 of 15 mentioned differences can be found in [25]. Figure 1. SchematicSchematic view view of of aerosol aerosol charging charging methods, methods, requiring requiring external external high high voltage voltage source: source: (a) (inductiona) induction method method in in aa 2-electrode 2-electrode system, system, (b) induction(b) induction method method in a 3-electrode in a 3-electrode system, (c )system, conduction (c) methodconduction in a method 2-electrode in a 2 system,-electrode (d) system, conduction (d) conduction method in amethod 3-electrode in a 3 system,-electrode (e) system, corona ( dischargee) corona method,discharge and method, (f) postdispersion and (f) postdispersion method. The method. main componentsThe main components of the system of the are: system N—conducting are: N— nozzleconducting acting nozzle as electrode; acting as S—streamelectrode; S of—stream liquid; of A—aerosol; liquid; A— T—target;aerosol; T— HV—hightarget; HV voltage—high voltage source; C—coronasource; C— electrode;corona electrode R—ring; R electrode;—ring electrode G—grid; G electrode.—grid electrode. To comparecompare didifferentfferent chargingcharging systems,systems, aa simplesimple capacitancecapacitance modelmodel andand electrostaticelectrostatic numericalnumerical simulations havehave been been proposed. proposed. Furthermore, Furthermore, the suggestedthe suggested concept concept seems seems to be su toffi cientbe sufficient to estimate to theestimate value the of Q value/m at lowof Q liquid/m at flowlow rates.liquid Theflow parameter rates. TheQ /parameterm provides Q an/m unambiguous provides an comparisonunambiguous of thecomparison individual of chargingthe individual systems charging from the systems point offrom view the of point their of effi viewciency. of their efficiency. InIn ourour research, research, both both arrangements arrangements are are analyzed analyzed only only in terms in terms of the of electrostatic the electrostatic field. In field. general, In q E thegeneral, induced the surfaceinduced charge surface density charge sidensityof conducting qsi of conducting objects is objects proportional is proportional to electric to field electricacting field on E thatacting object. on that For object. an object For an in aobject dielectric in a dielectric medium medium such as air,such for as which air, for relative which permittivityrelative permittivity may be q assumedmay be assumed as unitary as "unitaryr = 1, siεrcan = 1, beqsi givencan be by: given by: qsi푞푠푖= ="0 E휀0.퐸. (1)(1) To obtain the total charge Qi induced on the object (e.g., a droplet), one should integrate the To obtain the total charge Qi induced on the object (e.g., a droplet), one should integrate the charge overcharge the over entire the surface entire surfaces: s: Z Qi = qsids. (2) 푄 = ∫ 푞 d푠. (2) 푖 s 푠푖 푠 For a conducting object, the induced charge Qi is a result of the object–environment capacitance C and theFor resulting a conducting potential object, di fftheerence inducedV: charge Qi is a result of the object–environment capacitance C and the resulting potential difference V: Q = V C. (3) i · 푄 = 푉 ∙ 퐶. (3) 2. Capacitance Distribution in the Charging Systems푖 When spraying with water and aqueous solutions (e.g., pesticides), a droplet can be effectively 2. Capacitance Distribution in the Charging Systems treated as a conducting object. This is due to the fact that the droplet is affected by an electric field longerWhen than spraying its material with charge–transfer water and aqueous time solutions constant τ(e.g.[1,2, ].pesticides), For the mentioned a droplet liquids,can be effectively this time constanttreated as can a conducting be lower than object. 1 µs This [2]. As is due can beto seenthe fact from that Equation the droplet (3), the is affected electrical by capacitance an electric of field the systemlonger than used its during material the charging charge–transfer process istime the constant main factor τ [1,2] (i.e.,. apartFor the from mentioned the electric liquids, field relatedthis time to theconsta potentialnt can dibeff lowererence thanV) that 1 μs directly [2]. As influencescan be seen the from value Equation of the charge(3), the induced electrical on capacitance the droplet. of The the capacitancesystem used distributions during the charging in two- and process three-electrode is the main systems factor (i.e. are, schematicallyapart from the shown electric in field Figure related2. to the potential difference V) that directly influences the value of the charge induced on the droplet. Energies 2020, 13, x FOR PEER REVIEW 3 of 15 EnergiesThe capacitance2020, 13, 469 distributions in two- and three-electrode systems are schematically shown in Figure3 of 15 2. Figure 2. Capacitance distribution in a (a) 2-electrode system and (b) 3-electrode system. Note: T—target; Figure 2. Capacitance distribution in a (a) 2-electrode system and (b) 3-electrode system. Note: T— R—ring electrode; D—droplet; N—nozzle; C2nt—capacitance between nozzle and target in a 2-electrode target; R—ring electrode; D—droplet; N—nozzle; C2nt—capacitance between nozzle and target in a 2- system; C2dt—capacitance between droplet and target in 2-electrode system; C3nt—capacitance between electrode system; C2dt—capacitance between droplet and target in 2-electrode system; C3nt— nozzle and target in a 3-electrode system; C3nr—capacitance between nozzle and ring electrode in capacitance between nozzle and target in a 3-electrode system; C3nr—capacitance between nozzle and a 3-electrode system; C3dr—capacitance between droplet and ring electrode in a 3-electrode system; ring electrode in a 3-electrode system; C3dr—capacitance between droplet and ring electrode in a 3- C3dt—capacitance between droplet and target in a 3-electrode system; C3rt—capacitance between ring electrodeelectrode andsystem target; C in3dt a— 3-electrodecapacitance system. between
Details
-
File Typepdf
-
Upload Time-
-
Content LanguagesEnglish
-
Upload UserAnonymous/Not logged-in
-
File Pages15 Page
-
File Size-