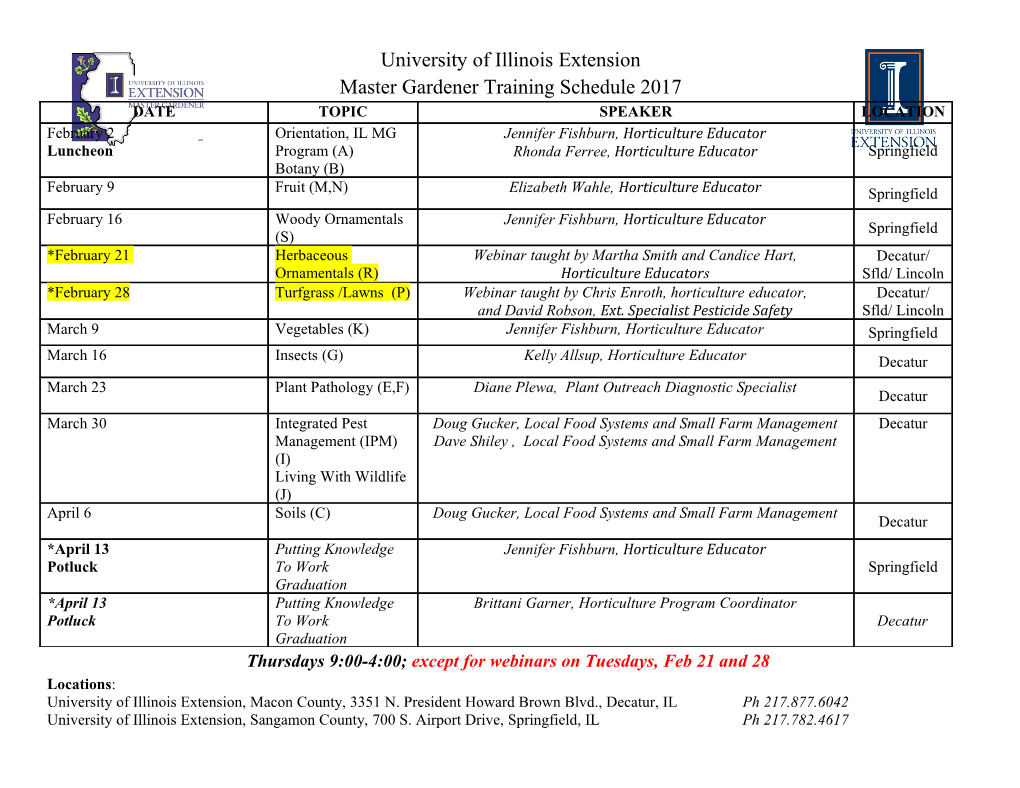
Metallurgical Analysis of Shell and Case Shot Artillery from the Civil War Battles of Pea Ridge and Wilson’s Creek Alicia L. Caporaso, Carl G. Carlson-Drexler, and Joel Masters ABSTRACT Interdisciplinary study in archaeology is important for leading up to the battles. This is important for understand- extracting new information from well-studied artifact ing foundry practices before and during the Civil War, as sources. The Civil War battles of Wilson’s Creek, Missouri arsenal records from this time are poor. in August 1861 and Pea Ridge, Arkansas in February 1862 provide archaeologists with hundreds of testable artillery remains. By utilizing metallurgical analyses in studying artillery fragments, the physical properties involved in their manufacture and use can be determined. The analysis shows that there is measurable variability in the metallurgy of Federal and Confederate ordnance. It also shows that in the western theater Federal ordnance manufacture was more uniform than Confederate manufacture. Introduction Battlefields as archaeological sites are a relatively common focus for study. Battlefield archaeology has been somewhat Figure 1. Confederate ordnance fragments from the battles of superficial, however, concentrating on actions and events Pea Ridge, Arkansas and Wilson’s Creek, Missouri. (Photo by that occurred with an assumption that artifact counts and Alicia Caporaso, 2003.) locations provide a full spectrum of data for interpreta- tion. While curating ordnance from the early Civil War battles of Wilson’s Creek and Pea Ridge, Douglas Scott of the National Park Service noticed that fragments of shell and case shot ordnance were relatively uniform in size and shape (Figures 1 and 2). Scott questioned whether or not it was possible to determine what processes created these artifacts. The following metallurgical tests may be useful in eliciting information to assist in answering this query: finite element analysis, optical and scanning electron microscopy, chemical profiling, and hardness testing. The results show that metallurgical analysis of military ordnance is a useful and informative tool that provides data unavailable through conventional archaeological methods. It not only aids in understanding their use and the results Figure 2. Federal ordnance fragments from the battles of Pea of their use on the battlefields, but also illuminates the Ridge, Arkansas and Wilson’s Creek, Missouri. (Photo by Alicia processes of manufacture and procurement in the events Caporaso, 2003.) TECHNICAL BRIEFS IN HISTORICAL ARCHAEOLOGY, 2008, 3: 15–24 METALLURGICAL ANALYSIS OF SHELL AND CASE SHOT ARTILLERY FROM CIVIL WAR BATTLES Gray Cast Iron graphite flakes within the matrix. The typical microstruc- ture is a matrix of pearlite (a layered mixture of ferrite By the time of the Civil War, metallurgists had appreciable and cementite) with pointed (Callister 2001:410) graphite understanding of the properties of cast iron. Artillerists flakes dispersed throughout.V ery slow cooling is likely recommended gray cast iron for ordnance use (Gibbon to produce considerable ferrite throughout the matrix 1860:171). It is likely that the choice factors were its (Lyman 1961:351). ease of casting and low cost (Lyman 1961:350; Callister Graphite shape is directly related to strength (Davis 2001:349). 1996:7). Of all the cast irons, gray iron has the lowest Civil War era metallurgists knew that gray cast iron has impact resistance (Lyman 1961:357, Davis 1996:45). high castability, good fluidity and expansion upon cooling Maximizing this characteristic in ordnance is ideal, as the in molds, the melting point is low (~1200°C), and the cast maximum amount of energy will be transferred to the form has strength in compression and weakness in tension projectile fragments. (Scott 1991). In addition, the metallurgist could produce All gray irons fail in a brittle manner. Fracture occurs a consistent product. along the lamellar graphite plates (Davis 1996:4) and at All gray irons contain iron, carbon, and silicon as well maximum compressive loads (Angus 1976:46). A cannon as appreciable amounts of phosphorus, sulfur, and manga- ball can be considered as a thick-walled spherical pressure nese. The free graphite in the material matrix is formed “as vessel and the failure as an impact overload exceeding the cast” and can take a number of different microstructures compressive strength. The mode of failure can be attributed (Bolton 1937:80–81). This is archaeologically useful; it can to thermal stress overload wherein the stress from a thermal be assumed that artillery produced in different foundries change (the charge) demands a specific change of dimen- and at different times will have varying microstructures. sion. As gray cast iron cannot expand plastically, the yield The following discussion will often refer to gray iron strength is exceeded, causing fracture (Davis 1996:347). as eutectic. A eutectic reaction is a reaction in which, upon Variations in graphite size and distribution cause varia- cooling, a liquid phase transforms isothermally and revers- tions in hardness readings across samples. The hardness of ibly into two mixed solid phases. The phases exist as alter- the metallic matrix is constant, however, (Lyman 1961:356). nating lamellae. In gray iron, the reaction is as follows: While Angus (1976:48) states that there is no relationship between hardness and tensile strength for cast iron, he does Fe C → 3Fe(α) + C 3 not relate hardness and compressive strength. Fe = iron Slight variations in the chemical make-up will alter me- C = carbon chanical properties of cast iron. Typical alloying elements α = ferrite in gray cast iron include: silicon and aluminum, which For gray cast iron, there are two important periods for increase graphitization, increase the ferrite-pearlite ratio, cooling. The first occurs between 1135°C and 1150°C, and lowers strength; nickel, copper, and tin, which increase when graphite is formed and the ratio of combined to graphitization, increase pearlite, and raises strength and graphitic carbon is fixed (Lyman 1961:351). The second is hardness; and chromium, molybdenum, tungsten, and at 650°C to 720°C when the matrix is determined (Angus vanadium, which decrease graphitization and increase 1976:36). Slow cooling favors graphite production while strength (Davis 1996:8). Silicon and nickel decrease hard- rapid cooling favors metastable ferrite and some free ness due to their tendency to increase graphitization, while cementite (iron carbide, Fe3C) or mottled iron (Davis phosphorus, manganese, sulphur, chromium, molybde- 1996:8; Lyman 1972:81). num, and vanadium increase hardness (Angus 1976:51). In all gray cast iron, the sulfur and manganese content must Metallurgical Properties be balanced according to the following (Davis 1996:34): %Mn ≥ 1.7%S + 0.3% Cooling rate and temperature determines the chemical Mn = manganese composition of ferrite and graphite and the shape of the S = sulfur 16 TECHNICAL BRIEFS IN HISTORICAL ARCHAEOLOGY ALICIA L. CAPORASO, CARL G. CARLSON-DREXLER, AND JOEL MASTERS The total carbon, phosphorus, and silicon content, as re- Case shot are similar in dimension to shells, but they are lated in the carbon equivalent equation: usually thin walled to contain the maximum number of bul- lets, and are filled with small lead or iron balls and a mixture CE = %C + (%Si + %P)/3 of sulfur pitch or asphalt (Thomas 1985:16). The bullets act CE = carbon equivalent as a support to the case and prevent breakage by the force of C = carbon the discharge from the gun (Gibbon 1860:164–165). Si = silicon When burst, the pieces of the shell are dispersed in P = phosphorus almost every direction. The number of fragments is di- establishes the solidification temperature and is related rectly related to the brittleness of the material (Gibbon to foundry characteristics of the alloy (Davis 1996:6). In 1860:163, 250). Theoretical and experimental evidence impact tests, high phosphorus content decreases energy to shows that the least amount of resistance, and crack initia- rupture (Angus 1976:82). Gray cast iron usually contains tion, propagates through the fuse ring. from 1.7% to 4.5% carbon and 1.0% to 3.0% silicon (Lyman 1961:349). The carbon equivalent value tells im- Exemplar mediately the eutectic state of the iron (Angus 1976:3). Addition of silicon increases the stability of ferrite On 1 April 1865, the steamboat Bertrand sank in the De Soto while decreasing the stability of carbides (cementite), and Bend of the Missouri River in Western Iowa en route from promotes graphitization, which adversely affects strength St. Louis to the mining territories of Montana. Among the by lowering the percentage of carbon required for the eu- cargo was 12-pounder mountain howitzer case artillery tectic reaction and raising the eutectic temperature, which in crates marked “CANNON SHELLS FOR MOUNTAIN can modify graphite distribution. Silicon also increases HOWITZER, 1 doz. SHELLS FIXED FEBR. 1865, 18 castability by lowering the casting’s total contraction (Davis FRICTION PRIMERS FROM ST. LOUIS ARSENAL” 1996:5–6; Bolton 1937:135–136). (Petsche 1974:98). These shells are of the same morphol- ogy and manufacture as the shell fragments in question, and Ordnance Manufacture can be used as exemplars for interior dimensions. Figure 3 shows an example of a howitzer case loaded with lead shot There are two types of artillery projectiles analyzed in this study: spherical shell and case shot. The sphere is a com- mon shape of artillery as it presents the minimum surface for a given volume reducing the effect of wind resistance. If it strikes an object in flight, it is less deflected from its course than any other form (Gibbon 1860:155). Both ordnance types were manufactured similarly. The mold was made of sand mixed with clay and water. The projectile was allowed to cool while in the mold, allowing for slow cooling of the metal in layers, commencing on the outside of the casting with rising of the ambient tempera- ture of the sand (Gibbon 1860:73, 170–171).
Details
-
File Typepdf
-
Upload Time-
-
Content LanguagesEnglish
-
Upload UserAnonymous/Not logged-in
-
File Pages10 Page
-
File Size-